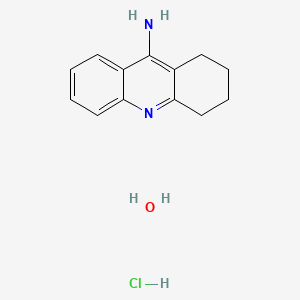
塩酸タクリジン一水和物
概要
説明
アセチルコリンエステラーゼによる加水分解の可逆的阻害によりコリン作動性シナプスにおけるアセチルコリン濃度を高めることで、コリン作動性機能を強化します . この化合物は、筋弛緩剤の効果に対抗する薬剤、呼吸促進剤、および他の中枢神経系疾患の治療薬として使用されてきました .
2. 製法
合成経路と反応条件: タクリン塩酸塩水和物は、さまざまな方法で合成できます。 持続可能なアプローチの1つは、深共融溶媒(DESs)をエアロビック条件下で使用し、98%の収率を達成することです . この方法は、揮発性有機化合物をより環境に優しい溶媒に置き換えるため、よりグリーンな代替手段となっています。 最適化されたプロトコルは、3グラムの基質に簡単にスケールアップでき、収率の低下なく、肝毒性が低減されたタクリン誘導体にまで成功裏に拡張できます .
工業的生産方法: 工業的には、タクリン塩酸塩水和物は、正確に秤量したタクリン塩酸塩を移動相に溶解し、移動相で定量的に希釈することにより、既知の濃度の溶液を得て製造されます .
3. 化学反応解析
反応の種類: タクリン塩酸塩水和物は、以下を含むさまざまな化学反応を起こします。
酸化: 特定の条件下で酸化される可能性があります。
還元: 還元反応によってその構造を変更できます。
置換: 置換反応によって、分子にさまざまな官能基を導入できます。
一般的な試薬と条件: これらの反応に使用される一般的な試薬には、酸化剤、還元剤、およびさまざまな触媒が含まれます。 条件は、目的の反応と生成物に応じて異なります。
主な生成物: これらの反応から生成される主な生成物には、さまざまなタクリン誘導体が含まれており、それらは異なる薬理学的特性と肝毒性の低減を有することができます .
科学的研究の応用
タクリン塩酸塩水和物は、幅広い科学研究用途を持っています。
化学: さまざまな化学研究において、コリンエステラーゼ阻害剤として使用されます。
生物学: コリンエステラーゼ阻害が生物系に及ぼす影響を研究するために使用されます。
作用機序
タクリン塩酸塩水和物は、コリン作動性機能を強化することでその効果を発揮します。 これは、アセチルコリンエステラーゼによる加水分解の可逆的阻害によりコリン作動性シナプスにおけるアセチルコリン濃度を高めることによって達成されます . 分子標的はコリンエステラーゼであり、関与する経路はコリン作動性伝達に関連しています .
類似化合物:
ドネペジル: アルツハイマー病の治療に使用される別のコリンエステラーゼ阻害剤。
リバスチグミン: 同様の作用機序を持つコリンエステラーゼ阻害剤。
ガランタミン: コリン作動性機能を強化するために使用される別の化合物。
独自性: タクリン塩酸塩水和物は、その独特の構造と、肝毒性が低減されたさまざまな誘導体を形成する能力のためにユニークです . 深共融溶媒の合成における使用は、よりグリーンな生産方法の可能性も示しています .
生化学分析
Biochemical Properties
Tacrine Hydrochloride Monohydrate is a parasympathomimetic, a reversible cholinesterase inhibitor that enhances cholinergic function by increasing the concentration of acetylcholine at cholinergic synapses through reversible inhibition of its hydrolysis by acetylcholinesterase .
Cellular Effects
Tacrine Hydrochloride Monohydrate exerts its effects on various types of cells, particularly neurons in the brain. It influences cell function by enhancing cholinergic function, which is crucial for memory and cognition .
Molecular Mechanism
The molecular mechanism of action of Tacrine Hydrochloride Monohydrate involves binding to acetylcholinesterase, inhibiting its activity, and thereby increasing the levels of acetylcholine in the brain . This results in enhanced cholinergic function, which is beneficial in conditions like Alzheimer’s disease where there is a deficiency of acetylcholine .
Temporal Effects in Laboratory Settings
It is known that the drug has a relatively short half-life, necessitating multiple daily doses .
Dosage Effects in Animal Models
In animal models, the effects of Tacrine Hydrochloride Monohydrate vary with dosage. High doses can lead to adverse effects such as hepatotoxicity, while lower doses are generally well-tolerated .
Metabolic Pathways
The major form of metabolism of Tacrine Hydrochloride Monohydrate is in the liver via hydroxylation of the benzylic carbon by Cytochrome P450 1A2. This forms the major metabolite 1-hydroxy-tacrine (velnacrine) which is still active .
Transport and Distribution
It is known that the drug can cross the blood-brain barrier, which allows it to exert its effects on the central nervous system .
Subcellular Localization
Given its mechanism of action, it is likely that it interacts with acetylcholinesterase at the synaptic cleft in neurons .
準備方法
Synthetic Routes and Reaction Conditions: Tacrine hydrochloride hydrate can be synthesized through various methods. One sustainable approach involves using deep eutectic solvents (DESs) under aerobic conditions, achieving a 98% yield . This method replaces volatile organic compounds with more eco-friendly solvents, making it a greener alternative. The optimized protocol scales easily to 3 grams of substrate without yield loss and extends successfully to tacrine derivatives with reduced hepatotoxicity .
Industrial Production Methods: In industrial settings, tacrine hydrochloride hydrate is produced by dissolving an accurately weighed quantity of tacrine hydrochloride in a mobile phase and diluting it quantitatively with the mobile phase to obtain a solution with a known concentration .
化学反応の分析
Types of Reactions: Tacrine hydrochloride hydrate undergoes various chemical reactions, including:
Oxidation: It can be oxidized under specific conditions.
Reduction: Reduction reactions can modify its structure.
Substitution: Substitution reactions can introduce different functional groups into the molecule.
Common Reagents and Conditions: Common reagents used in these reactions include oxidizing agents, reducing agents, and various catalysts. The conditions vary depending on the desired reaction and product.
Major Products: The major products formed from these reactions include various derivatives of tacrine, which can have different pharmacological properties and reduced hepatotoxicity .
類似化合物との比較
Donepezil: Another cholinesterase inhibitor used in the treatment of Alzheimer’s disease.
Rivastigmine: A cholinesterase inhibitor with a similar mechanism of action.
Galantamine: Another compound used to enhance cholinergic function.
Uniqueness: Tacrine hydrochloride hydrate is unique due to its specific structure and the ability to form various derivatives with reduced hepatotoxicity . Its use of deep eutectic solvents in synthesis also highlights its potential for greener production methods .
特性
IUPAC Name |
1,2,3,4-tetrahydroacridin-9-amine;hydrate;hydrochloride | |
---|---|---|
Source | PubChem | |
URL | https://pubchem.ncbi.nlm.nih.gov | |
Description | Data deposited in or computed by PubChem | |
InChI |
InChI=1S/C13H14N2.ClH.H2O/c14-13-9-5-1-3-7-11(9)15-12-8-4-2-6-10(12)13;;/h1,3,5,7H,2,4,6,8H2,(H2,14,15);1H;1H2 | |
Source | PubChem | |
URL | https://pubchem.ncbi.nlm.nih.gov | |
Description | Data deposited in or computed by PubChem | |
InChI Key |
PXGRMZYJAOQPNZ-UHFFFAOYSA-N | |
Source | PubChem | |
URL | https://pubchem.ncbi.nlm.nih.gov | |
Description | Data deposited in or computed by PubChem | |
Canonical SMILES |
C1CCC2=NC3=CC=CC=C3C(=C2C1)N.O.Cl | |
Source | PubChem | |
URL | https://pubchem.ncbi.nlm.nih.gov | |
Description | Data deposited in or computed by PubChem | |
Molecular Formula |
C13H17ClN2O | |
Source | PubChem | |
URL | https://pubchem.ncbi.nlm.nih.gov | |
Description | Data deposited in or computed by PubChem | |
DSSTOX Substance ID |
DTXSID80221711 | |
Record name | Tacrine hydrochloride monohydrate | |
Source | EPA DSSTox | |
URL | https://comptox.epa.gov/dashboard/DTXSID80221711 | |
Description | DSSTox provides a high quality public chemistry resource for supporting improved predictive toxicology. | |
Molecular Weight |
252.74 g/mol | |
Source | PubChem | |
URL | https://pubchem.ncbi.nlm.nih.gov | |
Description | Data deposited in or computed by PubChem | |
Solubility |
>37.9 [ug/mL] (The mean of the results at pH 7.4) | |
Record name | SID56424070 | |
Source | Burnham Center for Chemical Genomics | |
URL | https://pubchem.ncbi.nlm.nih.gov/bioassay/1996#section=Data-Table | |
Description | Aqueous solubility in buffer at pH 7.4 | |
CAS No. |
206658-92-6, 7149-50-0 | |
Record name | 1,2,3,4-Tetrahydro-9-acridinamine hydrochloride hydrate (1:?:?) | |
Source | CAS Common Chemistry | |
URL | https://commonchemistry.cas.org/detail?cas_rn=206658-92-6 | |
Description | CAS Common Chemistry is an open community resource for accessing chemical information. Nearly 500,000 chemical substances from CAS REGISTRY cover areas of community interest, including common and frequently regulated chemicals, and those relevant to high school and undergraduate chemistry classes. This chemical information, curated by our expert scientists, is provided in alignment with our mission as a division of the American Chemical Society. | |
Explanation | The data from CAS Common Chemistry is provided under a CC-BY-NC 4.0 license, unless otherwise stated. | |
Record name | Tacrine hydrochloride monohydrate | |
Source | ChemIDplus | |
URL | https://pubchem.ncbi.nlm.nih.gov/substance/?source=chemidplus&sourceid=0007149500 | |
Description | ChemIDplus is a free, web search system that provides access to the structure and nomenclature authority files used for the identification of chemical substances cited in National Library of Medicine (NLM) databases, including the TOXNET system. | |
Record name | Tacrine hydrochloride monohydrate | |
Source | EPA DSSTox | |
URL | https://comptox.epa.gov/dashboard/DTXSID80221711 | |
Description | DSSTox provides a high quality public chemistry resource for supporting improved predictive toxicology. | |
Record name | Tacrine | |
Source | European Chemicals Agency (ECHA) | |
URL | https://echa.europa.eu/information-on-chemicals | |
Description | The European Chemicals Agency (ECHA) is an agency of the European Union which is the driving force among regulatory authorities in implementing the EU's groundbreaking chemicals legislation for the benefit of human health and the environment as well as for innovation and competitiveness. | |
Explanation | Use of the information, documents and data from the ECHA website is subject to the terms and conditions of this Legal Notice, and subject to other binding limitations provided for under applicable law, the information, documents and data made available on the ECHA website may be reproduced, distributed and/or used, totally or in part, for non-commercial purposes provided that ECHA is acknowledged as the source: "Source: European Chemicals Agency, http://echa.europa.eu/". Such acknowledgement must be included in each copy of the material. ECHA permits and encourages organisations and individuals to create links to the ECHA website under the following cumulative conditions: Links can only be made to webpages that provide a link to the Legal Notice page. | |
Record name | TACRINE HYDROCHLORIDE MONOHYDRATE | |
Source | FDA Global Substance Registration System (GSRS) | |
URL | https://gsrs.ncats.nih.gov/ginas/app/beta/substances/MQ603P8SBL | |
Description | The FDA Global Substance Registration System (GSRS) enables the efficient and accurate exchange of information on what substances are in regulated products. Instead of relying on names, which vary across regulatory domains, countries, and regions, the GSRS knowledge base makes it possible for substances to be defined by standardized, scientific descriptions. | |
Explanation | Unless otherwise noted, the contents of the FDA website (www.fda.gov), both text and graphics, are not copyrighted. They are in the public domain and may be republished, reprinted and otherwise used freely by anyone without the need to obtain permission from FDA. Credit to the U.S. Food and Drug Administration as the source is appreciated but not required. | |
Retrosynthesis Analysis
AI-Powered Synthesis Planning: Our tool employs the Template_relevance Pistachio, Template_relevance Bkms_metabolic, Template_relevance Pistachio_ringbreaker, Template_relevance Reaxys, Template_relevance Reaxys_biocatalysis model, leveraging a vast database of chemical reactions to predict feasible synthetic routes.
One-Step Synthesis Focus: Specifically designed for one-step synthesis, it provides concise and direct routes for your target compounds, streamlining the synthesis process.
Accurate Predictions: Utilizing the extensive PISTACHIO, BKMS_METABOLIC, PISTACHIO_RINGBREAKER, REAXYS, REAXYS_BIOCATALYSIS database, our tool offers high-accuracy predictions, reflecting the latest in chemical research and data.
Strategy Settings
Precursor scoring | Relevance Heuristic |
---|---|
Min. plausibility | 0.01 |
Model | Template_relevance |
Template Set | Pistachio/Bkms_metabolic/Pistachio_ringbreaker/Reaxys/Reaxys_biocatalysis |
Top-N result to add to graph | 6 |
Feasible Synthetic Routes
試験管内研究製品の免責事項と情報
BenchChemで提示されるすべての記事および製品情報は、情報提供を目的としています。BenchChemで購入可能な製品は、生体外研究のために特別に設計されています。生体外研究は、ラテン語の "in glass" に由来し、生物体の外で行われる実験を指します。これらの製品は医薬品または薬として分類されておらず、FDAから任何の医療状態、病気、または疾患の予防、治療、または治癒のために承認されていません。これらの製品を人間または動物に体内に導入する形態は、法律により厳格に禁止されています。これらのガイドラインに従うことは、研究と実験において法的および倫理的な基準の遵守を確実にするために重要です。