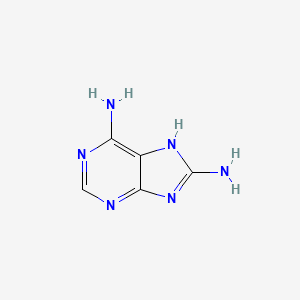
9H-Purine-6,8-diamine
概要
説明
準備方法
The synthesis of 9H-Purine-6,8-diamine typically involves the modification of purine derivatives. One common method includes the reaction of 2-amino-6-chloropurine with appropriate reagents to introduce the amino group at the 8-position. This process often requires specific reaction conditions such as the use of dimethylformamide (DMF) as a solvent and the presence of phase transfer catalysts . Industrial production methods may involve large-scale synthesis using similar routes but optimized for higher yields and purity.
化学反応の分析
Nucleophilic Substitution Reactions
The primary amino groups at positions 6 and 8 of the purine core are highly reactive toward nucleophilic substitution. For example:
-
N6-Substitution : Reaction of 2-amino-6-chloropurine with amines in dimethylformamide (DMF) at 80°C yields N6-substituted derivatives. Cyclopropylamine, aniline derivatives, and trifluoroethoxy-substituted phenylamines have been successfully introduced at this position, with yields ranging from 78% to 90% .
-
N8-Substitution : Alkylation at N8 using methyl iodide under basic conditions produces N8-methyl derivatives. This reaction is critical for modifying steric and electronic properties of the purine scaffold.
Table 1: Substitution Reactions of 9H-Purine-6,8-diamine
Oxidation and Reduction
The purine ring undergoes redox transformations under controlled conditions:
-
Oxidation : Treatment with potassium permanganate (KMnO₄) or hydrogen peroxide (H₂O₂) oxidizes the purine scaffold to form 2’-deoxyinosine analogs, which are structurally related to natural nucleosides.
-
Reduction : Sodium borohydride (NaBH₄) reduces the imine bonds in the purine core, yielding dihydro derivatives. This reaction is pivotal for probing electronic effects on biological activity.
Table 2: Redox Reactions and Products
Reaction | Reagents | Product | Key Application |
---|---|---|---|
Oxidation | KMnO₄/H₂O₂ | 2’-Deoxyinosine derivatives | Nucleoside analog synthesis |
Reduction | NaBH₄/LiAlH₄ | Dihydro-9H-purine-6,8-diamine | Mechanistic studies |
Functionalization via Cross-Coupling
Palladium-catalyzed cross-coupling reactions enable the introduction of aryl and heteroaryl groups:
-
Suzuki-Miyaura Coupling : Reaction with arylboronic acids in the presence of Pd(PPh₃)₄ produces 6-arylpurines. For instance, coupling with 5-bromo-2-methoxyphenylboronic acid yields 6-(5-bromo-2-methoxyphenyl)-9H-purine-6,8-diamine (70% yield) .
-
Buchwald-Hartwig Amination : This method facilitates C–N bond formation at position 8, enabling the synthesis of diarylamine-substituted purines .
Acylation and Alkylation
The exocyclic amines undergo acylation and alkylation to enhance solubility or biological targeting:
-
Acylation : Treatment with acyl chlorides (e.g., acetyl chloride) in the presence of triethylamine introduces acyl groups at N6 or N8, producing amide derivatives.
-
Alkylation : Alkyl halides react with the amino groups under basic conditions to form N-alkylated purines, which are intermediates in kinase inhibitor development .
Mechanistic Insights
-
Substitution Kinetics : The N6 position exhibits higher reactivity than N8 due to reduced steric hindrance, as shown in computational studies .
-
Regioselectivity : Electron-withdrawing substituents on the purine ring direct nucleophilic attack to specific positions, enabling controlled functionalization .
Stability and Reactivity Trends
-
pH Sensitivity : The compound undergoes hydrolysis under strongly acidic (pH < 2) or basic (pH > 10) conditions, limiting its use in aqueous media.
-
Thermal Stability : Decomposition occurs above 300°C, making it unsuitable for high-temperature reactions.
科学的研究の応用
Scientific Research Applications
The applications of 9H-Purine-6,8-diamine can be categorized into several key areas:
Pharmaceutical Development
- Antitumor Activity : Research indicates that derivatives of this compound exhibit potential antitumor properties. These compounds are being explored for their ability to inhibit DNA topoisomerase II, an enzyme crucial for DNA replication and transcription. Inhibiting this enzyme can lead to the suppression of cancer cell proliferation .
- Antiviral Properties : The compound has also shown promise as an antiviral agent. Its structural characteristics allow it to interact effectively with viral enzymes, potentially leading to the development of new antiviral therapies.
Biochemical Research
- Enzyme Inhibition : this compound serves as a valuable tool in studying enzyme mechanisms. It has been utilized in experiments aimed at understanding the role of purines in cellular processes, particularly in relation to DNA and RNA synthesis.
- Chlorination Studies : Recent studies have focused on the chlorination reactions involving purines. The reactivity of this compound under chlorinating conditions has been analyzed to understand its role in generating chlorinated nucleobases, which are implicated in genetic mutations and carcinogenesis .
Medicinal Chemistry
- Lead Compound for Drug Design : Due to its biological activity and structural features, this compound is considered a lead compound for developing new therapeutic agents targeting various diseases, including proliferative disorders and viral infections .
Case Studies
Several case studies highlight the applications of this compound:
Case Study 1: Antitumor Activity
A study published in a pharmaceutical journal demonstrated that a derivative of this compound effectively inhibited the growth of cancer cells in vitro. The mechanism involved the selective inhibition of DNA topoisomerase II, leading to increased apoptosis in tumor cells .
Case Study 2: Antiviral Research
In another investigation focusing on viral infections, researchers synthesized several analogs of this compound. These compounds were tested against common viruses such as HIV and influenza. Results indicated that some derivatives exhibited significant antiviral activity by disrupting viral replication pathways.
作用機序
The mechanism of action of 9H-Purine-6,8-diamine involves its interaction with specific molecular targets. For instance, it can inhibit enzymes such as dihydrofolate reductase (DHFR), which is crucial for DNA synthesis. This inhibition leads to the disruption of nucleotide synthesis, ultimately causing cell cycle arrest and apoptosis in cancer cells . The compound’s effects are mediated through pathways involving lysosomes and mitochondria, indicating a complex mechanism of inducing cell death .
類似化合物との比較
9H-Purine-6,8-diamine can be compared with other purine derivatives such as:
2,6-Diaminopurine: Similar in structure but with different substitution patterns, leading to varied biological activities.
6-Morpholin-4-yl-9H-purine: Another purine derivative with distinct pharmacological properties.
8-Aminoguanine: Shares the amino group at the 8-position but differs in other structural aspects.
The uniqueness of this compound lies in its specific substitution pattern, which imparts unique chemical and biological properties, making it a valuable compound for research and development in various scientific fields.
生物活性
9H-Purine-6,8-diamine, a purine derivative, has garnered attention for its diverse biological activities. This article synthesizes findings from various studies to present a comprehensive overview of its biological properties, mechanisms of action, and potential therapeutic applications.
Chemical Structure and Synthesis
This compound is characterized by its purine base structure with amino groups at the 6 and 8 positions. The synthesis of this compound often involves modifications of existing purine derivatives to enhance biological activity. For instance, the introduction of various substituents at the 2, 6, or 8 positions can significantly influence its pharmacological properties.
Anti-Inflammatory Effects
Recent studies have demonstrated that derivatives of this compound exhibit notable anti-inflammatory properties. For example, a novel derivative was shown to inhibit nitric oxide production in LPS-induced macrophages with an IC50 of 6.4 μM, outperforming resveratrol (IC50: 26.4 μM) in reducing pro-inflammatory cytokines such as IL-6 and TNF-α . This activity is mediated through the disruption of the TLR4–MyD88 signaling pathway, which is critical in inflammatory responses.
Table 1: Anti-Inflammatory Activity of Purine Derivatives
Compound | IC50 (μM) | Cytokines Inhibited | Mechanism of Action |
---|---|---|---|
9H-Purine Derivative | 6.4 | IL-6, TNF-α | TLR4–MyD88/NF-κB pathway inhibition |
Resveratrol | 26.4 | IL-6, TNF-α | NF-κB pathway modulation |
Antidiabetic Properties
Another significant area of research involves the antidiabetic effects of purine derivatives. A study highlighted an 8-purine derivative that selectively inhibited DPP-4, an enzyme involved in glucose metabolism. This compound demonstrated an IC50 value of 0.1 nM and effectively reduced hyperglycemia in Zücker obese rats . The reduction in HbA1c levels further underscores its potential as a therapeutic agent for diabetes management.
Table 2: Antidiabetic Activity of Purine Derivatives
Compound | IC50 (nM) | Effect on HbA1c (%) | Model Used |
---|---|---|---|
8-Purine Derivative | 0.1 | Decreased from 8.5 to 7.3 | Zücker obese rats |
The biological activity of this compound derivatives can be attributed to several mechanisms:
- Inhibition of Enzymatic Activity : Many derivatives act as ATP competitive inhibitors or inhibit topoisomerase II, which is crucial for DNA replication and repair .
- Modulation of Signaling Pathways : The compounds have been shown to interfere with key signaling pathways involved in inflammation and cell proliferation.
- Antioxidant Properties : Some studies suggest that certain purine derivatives may exhibit antioxidant effects, which could mitigate oxidative stress-related damage .
Case Studies
Several case studies have explored the therapeutic potential of purine derivatives:
Q & A
Basic Research Questions
Q. Q1. What established synthetic routes are available for 9H-Purine-6,8-diamine, and how is its structural integrity validated?
Answer: Synthesis typically involves condensation reactions with amine precursors under controlled pH and temperature. For example, analogous purine derivatives are synthesized via Skraup-like reactions using sulfuric acid as a catalyst, with nitrobenzene as an oxidizing agent . Post-synthesis, structural validation employs:
- Mass spectrometry (ESI) to confirm molecular weight .
- Infrared spectroscopy (IR) to identify functional groups (e.g., NH₂ stretches at ~3300 cm⁻¹) .
- X-ray crystallography for resolving bond angles and torsional strain, as demonstrated in platinum-purine complexes .
Q. Q2. What thermodynamic properties of this compound are critical for stability assessment in experimental conditions?
Answer: Key thermodynamic parameters include:
- Enthalpy of formation (ΔfH°solid): Reported values vary (e.g., 169.4 ± 2.7 kJ/mol via calorimetry ).
- Heat capacity (Cp): Critical for reaction optimization (e.g., 106.9 J/mol·K at 298.15 K ).
- Ionization energy (IE): ~9.52 eV (vertical PE measurement) informs redox behavior in aqueous systems .
Table 1: Thermodynamic Data for 9H-Purine Derivatives
Property | Value | Method | Reference |
---|---|---|---|
ΔfH°solid (kJ/mol) | 169.4 ± 2.7 | Calorimetry | |
Cp (J/mol·K) | 106.9 | DH method | |
Ionization Energy (eV) | 9.52 ± 0.03 | Photoelectron |
Advanced Research Questions
Q. Q3. How can researchers resolve contradictions in reported thermodynamic data for this compound?
Answer: Discrepancies in ΔfH° values (e.g., 169.4 vs. 2238.05 kJ/mol ) arise from methodological differences:
- Calorimetry (Ccr) vs. combustion bomb (Ccb) techniques.
- Replication strategies: Use high-purity samples, standardized protocols (e.g., NIST guidelines ), and error analysis (e.g., ±2.7 kJ/mol uncertainty ).
- Computational validation: Compare experimental ΔfH° with DFT-calculated values to identify systematic errors.
Q. Q4. What experimental design considerations optimize the synthesis of this compound derivatives?
Answer: Critical factors include:
- Catalyst selection: Acidic conditions (e.g., p-TSA) enhance reaction rates, as seen in perimidine syntheses .
- Solvent polarity: Ethanol or DMF improves solubility of amine intermediates .
- Temperature control: Reactions above 130°C risk decomposition; lower temperatures favor selectivity .
Table 2: Synthesis Optimization Parameters
Parameter | Optimal Range | Impact on Yield | Reference |
---|---|---|---|
Reaction Temperature | 120–130°C | Prevents side reactions | |
Catalyst (p-TSA) | 2 mol% | Maximizes efficiency | |
Solvent | Ethanol | Enhances solubility |
Q. Q5. How can computational models predict the reactivity of this compound in nucleophilic substitution reactions?
Answer:
- DFT calculations: Model charge distribution at C6 and C8 positions to identify nucleophilic attack sites.
- Transition state analysis: Use software (e.g., Gaussian) to simulate activation energies, validated against experimental kinetics .
- Electron localization: Compare HOMO-LUMO gaps (e.g., 9.7 eV IE ) to assess electron donor/acceptor capacity.
Q. Methodological Guidance for Data Analysis
Q. Q6. What statistical approaches are recommended for analyzing contradictory spectral data in purine derivatives?
Answer:
- Multivariate analysis: Apply PCA to IR or NMR datasets to isolate outliers .
- Error propagation: Calculate combined uncertainties for overlapping peaks (e.g., NH₂ vs. OH stretches).
- Cross-validation: Compare results across techniques (e.g., X-ray vs. computational bond lengths ).
Q. Q7. How should researchers critically evaluate synthetic routes for scalability in academic settings?
Answer:
- Green chemistry metrics: Assess E-factor and atom economy, avoiding hazardous solvents (e.g., nitrobenzene ).
- Reproducibility: Document reaction conditions (e.g., stirring time, drying methods) per extended essay standards .
- Yield vs. purity trade-offs: Prioritize chromatography (e.g., silica gel ) over recrystallization for complex mixtures.
特性
IUPAC Name |
7H-purine-6,8-diamine | |
---|---|---|
Source | PubChem | |
URL | https://pubchem.ncbi.nlm.nih.gov | |
Description | Data deposited in or computed by PubChem | |
InChI |
InChI=1S/C5H6N6/c6-3-2-4(9-1-8-3)11-5(7)10-2/h1H,(H5,6,7,8,9,10,11) | |
Source | PubChem | |
URL | https://pubchem.ncbi.nlm.nih.gov | |
Description | Data deposited in or computed by PubChem | |
InChI Key |
PFUVOLUPRFCPMN-UHFFFAOYSA-N | |
Source | PubChem | |
URL | https://pubchem.ncbi.nlm.nih.gov | |
Description | Data deposited in or computed by PubChem | |
Canonical SMILES |
C1=NC(=C2C(=N1)N=C(N2)N)N | |
Source | PubChem | |
URL | https://pubchem.ncbi.nlm.nih.gov | |
Description | Data deposited in or computed by PubChem | |
Molecular Formula |
C5H6N6 | |
Source | PubChem | |
URL | https://pubchem.ncbi.nlm.nih.gov | |
Description | Data deposited in or computed by PubChem | |
DSSTOX Substance ID |
DTXSID20402507 | |
Record name | 1H-Purine-6,8-diamine | |
Source | EPA DSSTox | |
URL | https://comptox.epa.gov/dashboard/DTXSID20402507 | |
Description | DSSTox provides a high quality public chemistry resource for supporting improved predictive toxicology. | |
Molecular Weight |
150.14 g/mol | |
Source | PubChem | |
URL | https://pubchem.ncbi.nlm.nih.gov | |
Description | Data deposited in or computed by PubChem | |
CAS No. |
28128-33-8 | |
Record name | 1H-Purine-6,8-diamine | |
Source | DTP/NCI | |
URL | https://dtp.cancer.gov/dtpstandard/servlet/dwindex?searchtype=NSC&outputformat=html&searchlist=21698 | |
Description | The NCI Development Therapeutics Program (DTP) provides services and resources to the academic and private-sector research communities worldwide to facilitate the discovery and development of new cancer therapeutic agents. | |
Explanation | Unless otherwise indicated, all text within NCI products is free of copyright and may be reused without our permission. Credit the National Cancer Institute as the source. | |
Record name | 1H-Purine-6,8-diamine | |
Source | EPA DSSTox | |
URL | https://comptox.epa.gov/dashboard/DTXSID20402507 | |
Description | DSSTox provides a high quality public chemistry resource for supporting improved predictive toxicology. | |
Retrosynthesis Analysis
AI-Powered Synthesis Planning: Our tool employs the Template_relevance Pistachio, Template_relevance Bkms_metabolic, Template_relevance Pistachio_ringbreaker, Template_relevance Reaxys, Template_relevance Reaxys_biocatalysis model, leveraging a vast database of chemical reactions to predict feasible synthetic routes.
One-Step Synthesis Focus: Specifically designed for one-step synthesis, it provides concise and direct routes for your target compounds, streamlining the synthesis process.
Accurate Predictions: Utilizing the extensive PISTACHIO, BKMS_METABOLIC, PISTACHIO_RINGBREAKER, REAXYS, REAXYS_BIOCATALYSIS database, our tool offers high-accuracy predictions, reflecting the latest in chemical research and data.
Strategy Settings
Precursor scoring | Relevance Heuristic |
---|---|
Min. plausibility | 0.01 |
Model | Template_relevance |
Template Set | Pistachio/Bkms_metabolic/Pistachio_ringbreaker/Reaxys/Reaxys_biocatalysis |
Top-N result to add to graph | 6 |
Feasible Synthetic Routes
試験管内研究製品の免責事項と情報
BenchChemで提示されるすべての記事および製品情報は、情報提供を目的としています。BenchChemで購入可能な製品は、生体外研究のために特別に設計されています。生体外研究は、ラテン語の "in glass" に由来し、生物体の外で行われる実験を指します。これらの製品は医薬品または薬として分類されておらず、FDAから任何の医療状態、病気、または疾患の予防、治療、または治癒のために承認されていません。これらの製品を人間または動物に体内に導入する形態は、法律により厳格に禁止されています。これらのガイドラインに従うことは、研究と実験において法的および倫理的な基準の遵守を確実にするために重要です。