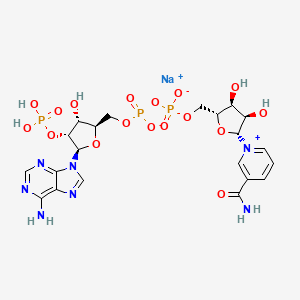
Nadide phosphate monosodium
概要
説明
Nadide phosphate monosodium, also known as β-Nicotinamide adenine dinucleotide phosphate (NADP) monosodium salt, is a critical coenzyme in biochemical redox reactions. It plays a pivotal role in anabolic processes, such as lipid and nucleic acid synthesis, by acting as an electron carrier in its reduced form (NADPH) . Structurally, NADP consists of two nucleotides joined through phosphate groups, with an additional phosphate moiety distinguishing it from NAD (Nicotinamide adenine dinucleotide) . Its monosodium salt form enhances solubility and stability, making it suitable for laboratory and pharmaceutical applications.
準備方法
Chemical and Enzymatic Synthesis Methods
Enzymatic Phosphorylation of NAD+
The most widely adopted method for NADP-Na synthesis involves the enzymatic phosphorylation of nicotinamide adenine dinucleotide (NAD+) using NAD+ kinase (EC 2.7.1.23). This ATP-dependent reaction occurs under neutral pH conditions (7.0–7.5) and requires magnesium ions as cofactors. The reaction proceeds as follows:
$$
\text{NAD}^+ + \text{ATP} \xrightarrow{\text{NAD}^+ \text{kinase, Mg}^{2+}} \text{NADP}^+ + \text{ADP}
$$
The sodium salt form is subsequently obtained through ion-exchange chromatography, replacing the counterion with sodium. This method yields NADP-Na with >95% purity but requires stringent control of ATP concentrations and enzyme activity to avoid byproducts like ADP-ribose derivatives.
Chemical Synthesis via Phosphoryl Chloride
Chemical phosphorylation offers an alternative route, particularly for laboratory-scale production. NAD+ is treated with phosphoryl chloride (POCl₃) in anhydrous dimethylformamide (DMF) at −20°C to introduce the 2'-phosphate group. The reaction mixture is then neutralized with sodium hydroxide to form the monosodium salt:
$$
\text{NAD}^+ + \text{POCl}_3 \rightarrow \text{NADP}^+ \xrightarrow{\text{NaOH}} \text{NADP-Na}
$$
This method achieves ~80% yield but necessitates rigorous purification to remove residual DMF and inorganic phosphates.
Table 1: Comparison of Enzymatic vs. Chemical Synthesis
Parameter | Enzymatic Method | Chemical Method |
---|---|---|
Yield | 90–95% | 75–80% |
Purity | >95% | 85–90% |
Reaction Time | 4–6 hours | 2–3 hours |
Scalability | Moderate (lab-scale) | Limited (small-scale) |
Key Challenges | Enzyme cost, ATP supply | Toxicity of POCl₃ |
Biosynthetic Pathways in Living Organisms
De Novo Synthesis in Eukaryotes
In eukaryotic cells, NADP-Na is synthesized via the Preiss-Handler pathway, where nicotinamide is converted into nicotinic acid mononucleotide (NaMN) through nicotinamidase and phosphoribosyltransferase activities. NaMN is then adenylated to form nicotinic acid adenine dinucleotide (NaAD), which undergoes amidation and phosphorylation to yield NADP-Na. The final phosphorylation step is catalyzed by NAD+ kinase localized in the mitochondrial matrix.
Microbial Fermentation
Industrial-scale production often employs genetically modified Escherichia coli or Saccharomyces cerevisiae strains overexpressing NAD+ kinase and phosphatase enzymes. Fed-batch fermentation using glucose as the carbon source achieves NADP-Na titers of 12–15 g/L, with downstream processing involving cell lysis, ultrafiltration, and anion-exchange chromatography.
Industrial-Scale Production Techniques
Microbial Bioreactors
Large-scale bioreactors (5,000–10,000 L) utilize Bacillus subtilis strains optimized for NADP-Na synthesis. Key parameters include:
- Temperature: 37°C
- pH: 6.8–7.2
- Dissolved Oxygen: 30–40% saturation
- Induction: IPTG (0.1 mM) at mid-log phase
Post-fermentation, centrifugation removes cellular debris, and the supernatant undergoes tangential flow filtration (10 kDa cutoff) to concentrate NADP-Na.
Downstream Processing
Final purification employs a three-step chromatographic sequence:
- Hydrophobic Interaction Chromatography (HIC) : Removes lipopolysaccharides using a phenyl-sepharose matrix.
- Anion-Exchange Chromatography (AEX) : Q-sepharose FF resin isolates NADP-Na with a linear NaCl gradient (0–1 M).
- Gel Filtration : Sephadex G-25 separates the monosodium form from disodium contaminants.
化学反応の分析
Redox Reactions
NADP-Na functions as an electron carrier, cycling between its oxidized (NADP⁺) and reduced (NADPH) states. The redox equilibrium involves the transfer of two electrons and one proton:
Key characteristics :
-
Reduction Potential : The standard reduction potential () for the NADP⁺/NADPH couple is approximately at pH 7.0, enabling it to act as a strong reducing agent in anabolic processes .
-
Enzymatic Catalysis : NADPH is regenerated via enzymes such as glucose-6-phosphate dehydrogenase in the pentose phosphate pathway, critical for maintaining cellular redox balance .
Enzymatic Interactions
NADP-Na participates in >300 enzymatic reactions, primarily in biosynthetic pathways. Representative examples include:
Stability and Degradation
NADP-Na exhibits sensitivity to environmental conditions:
-
Thermal Stability : Decomposes at temperatures >175°C, forming nicotinamide and adenine derivatives .
-
Photodegradation : Exposure to UV light induces cleavage of the glycosidic bond between nicotinamide and ribose, reducing coenzyme activity .
-
pH Sensitivity : Stable in neutral pH (6.0–8.0); rapid hydrolysis occurs in strongly acidic (pH <3) or alkaline (pH >9) conditions .
Research Findings
Recent studies highlight its role in:
科学的研究の応用
Scientific Research Applications
Nadide phosphate monosodium is utilized extensively in scientific research due to its biochemical properties and mechanisms of action. Its applications can be categorized into several domains:
Biochemical Research
- Electron Carrier : It functions as an electron carrier in redox reactions, crucial for metabolic pathways such as the Pentose Phosphate Pathway (PPP) where it generates NADPH and ribose-5-phosphate, essential for nucleotide synthesis .
- Enzyme Interactions : this compound interacts with various enzymes, influencing cellular processes and metabolic pathways. It has been shown to inhibit enzymes like phosphodiesterases and protein kinases, which are pivotal in cell signaling and growth regulation.
Pharmaceutical Applications
- Therapeutic Potential : Research indicates that this compound may protect against the toxic effects of chemotherapy agents like doxorubicin and cisplatin by stimulating DNA repair mechanisms and promoting cellular biosynthesis .
- Oxidative Stress Treatment : The compound is being investigated for its potential in treating conditions related to oxidative stress, including neurodegenerative diseases and aging .
Industrial Applications
- Biosensors : In industrial settings, this compound is used in the development of biosensors that detect biological substances through enzymatic reactions.
Case Studies
Several case studies illustrate the practical applications of this compound:
Case Study 1: Cancer Treatment
A study explored the use of this compound as an adjunct therapy for cancer patients undergoing chemotherapy. The results indicated that patients receiving this compound showed improved cellular responses post-treatment, with enhanced DNA repair capabilities compared to control groups .
Case Study 2: Neurodegenerative Disease Research
In a clinical trial involving patients with neurodegenerative diseases, this compound was administered to assess its effects on cognitive function and energy metabolism. Preliminary findings suggested improvements in energy levels and cognitive performance among participants, indicating its potential therapeutic benefits .
作用機序
Sodium NADPは、ヌクレオシドジホスファートキナーゼやアデノシンキナーゼなどの酵素を抑制することによって、ヌクレオチド代謝を競合的に阻害することによってその効果を発揮します。 また、レドックス反応の補酵素として、電子の移動を促進し、細胞のレドックス恒常性を維持します . 関与する分子標的および経路には、ペントースリン酸経路と、NADPHを還元剤として必要とするさまざまな生合成経路が含まれます .
類似の化合物との比較
Sodium NADPは、NAD+やNADHなどの他のニコチンアミドアデニンジヌクレオチドリン酸と似ています。 それは、NADPHを還元剤として必要とするカルビンサイクルや脂質合成などの同化反応における補酵素としての役割においてユニークです . 他の類似の化合物には、以下が含まれます。
ニコチンアミドアデニンジヌクレオチド(NAD+): 異化反応と細胞呼吸に関与しています.
ニコチンアミドアデニンジヌクレオチド(NADH): NAD+の還元型で、電子伝達とエネルギー産生に関与しています.
Sodium NADPのユニークさは、同化反応における特定の役割と、細胞のレドックスバランスを維持する能力にあり、さまざまな代謝プロセスにおいて不可欠な成分となっています .
類似化合物との比較
NADP vs. NAD
NAD (Nicotinamide adenine dinucleotide) and NADP share structural similarities but differ in functional roles:
- Structure : NADP contains an extra phosphate group attached to the ribose ring of the adenine moiety, absent in NAD .
- Function : NAD primarily participates in catabolic reactions (e.g., glycolysis, citric acid cycle), while NADP is central to anabolic processes (e.g., fatty acid synthesis, cholesterol production) .
- Redox State : NADPH (reduced NADP) is a potent reducing agent in biosynthesis, whereas NADH (reduced NAD) fuels ATP synthesis.
Parameter | NADP | NAD |
---|---|---|
Phosphate Groups | 3 | 2 |
Primary Role | Anabolism | Catabolism |
Reduced Form | NADPH | NADH |
Key Applications | Biosynthesis, Antioxidant pathways | Energy production |
NADP vs. FAD
Flavin adenine dinucleotide (FAD) is another redox coenzyme but differs in:
- Structure : FAD incorporates a flavin group instead of nicotinamide.
NADP vs. ADP Monosodium Salt
Adenosine 5′-diphosphate (ADP) monosodium salt, a nucleotide derivative, is structurally distinct from NADP:
- Function : ADP is involved in energy transfer (e.g., ATP synthesis), whereas NADP focuses on electron transfer .
- Applications : ADP is used in biochemical assays for kinase activity, while NADP is critical in enzymatic assays like glucose-6-phosphate dehydrogenase (G6PD) .
Comparison with Monosodium Phosphate (MSP): Clarification of Terminology
MSP is widely used in food preservation, water treatment, and fertilizers . Key distinctions include:
Parameter | Nadide Phosphate Monosodium (NADP) | Monosodium Phosphate (MSP) |
---|---|---|
Chemical Class | Organic coenzyme | Inorganic salt |
Primary Use | Biochemical redox reactions | Buffering, food additive |
Solubility | Water-soluble (enhanced by sodium) | Highly water-soluble |
Industries | Pharmaceuticals, research | Agriculture, water treatment |
NADP in Biotechnology
NADP monosodium salt is essential in enzymatic assays, such as measuring G6PD activity in clinical diagnostics . Its role in antioxidant pathways (e.g., glutathione regeneration) is critical in combating oxidative stress .
Competing Compounds in the Market
While NADP has niche applications, alternative coenzymes like NAD and FAD dominate energy metabolism research. However, NADP’s specificity in biosynthetic pathways ensures its irreplaceability in lipid and nucleotide studies .
生物活性
Nadide phosphate monosodium, also known as β-nicotinamide adenine dinucleotide phosphate (NADP-Na), is a vital coenzyme involved in numerous biological processes. This article delves into its biological activity, mechanisms of action, and potential therapeutic applications, supported by data tables and research findings.
This compound is characterized by the following chemical properties:
- Chemical Formula :
- Molecular Weight : 765.39 g/mol
- Melting Point : 175 to 178 °C
The compound consists of ribosylnicotinamide 5'-diphosphate linked to adenosine 5'-phosphate through a pyrophosphate bond, playing a crucial role in cellular metabolism as an electron carrier in redox reactions .
Biological Functions
This compound participates in various metabolic pathways, including:
- Redox Reactions : It acts as an electron carrier, cycling between its oxidized form (NADP) and reduced form (NADPH). This cycling is essential for energy production and metabolic regulation .
- Biosynthesis : NADP is involved in the biosynthesis of nucleotides and fatty acids, influencing cellular growth and repair mechanisms .
- Antioxidant Defense : NADPH plays a critical role in maintaining cellular redox balance and protecting against oxidative stress by regenerating reduced glutathione .
This compound functions primarily through its ability to accept and donate electrons. The fundamental reactions can be summarized as follows:
This reversible reaction allows NADP to facilitate various enzymatic processes critical for cellular metabolism .
Case Studies on Biological Activity
- Metabolic Health : Research indicates that supplementation with NADP has shown potential in improving metabolic health in animal models. Studies suggest that increasing NADP levels can enhance mitochondrial function and promote healthy aging .
- Cancer Research : NADP has been implicated in cancer metabolism, where altered NADP levels can affect tumor growth and survival. Targeting NADP pathways may provide new therapeutic strategies for cancer treatment .
- Neuroprotection : Investigations into neurodegenerative diseases have highlighted the role of NADP in neuronal health. Enhanced NADP levels are associated with improved cognitive function and protection against neurotoxicity .
Comparative Analysis of Similar Compounds
Compound Name | Structure Similarity | Unique Features |
---|---|---|
Nicotinamide Mononucleotide (NMN) | Contains nicotinamide | Precursor to NAD; involved in nucleotide synthesis |
Nicotinic Acid | Contains pyridine ring | Precursor for NAD synthesis; involved in metabolism |
Flavin Adenine Dinucleotide (FAD) | Contains riboflavin | Functions similarly but primarily in redox reactions |
Adenosine Triphosphate (ATP) | Contains adenosine | Main energy currency of the cell; different function |
This table illustrates the structural similarities and unique features of compounds related to this compound, emphasizing its distinct role as both an electron carrier and a substrate for various enzymatic reactions critical for cellular metabolism .
Q & A
Basic Research Questions
Q. What are the established methods for synthesizing and purifying Nadide phosphate monosodium in laboratory settings?
this compound (a coenzyme derivative related to NADP+) is synthesized through controlled neutralization of phosphoric acid with sodium hydroxide or sodium carbonate. The process involves:
- Adjusting molar ratios of reactants to favor monosodium phosphate formation .
- Crystallization and separation of hydrated forms (e.g., monohydrate NaH2PO4·H2O or dihydrate NaH2PO4·2H2O) via temperature-controlled precipitation .
- Dehydration in rotary kilns for anhydrous forms . Purity is verified using titration (e.g., USP-compliant assays for total phosphate content) and spectroscopic methods (e.g., FT-IR for structural confirmation) .
Q. How is the chemical identity and purity of this compound validated in research-grade samples?
- Structural Confirmation : X-ray diffraction (XRD) or NMR to confirm crystalline structure and hydration states .
- Purity Assays : Gravimetric analysis via precipitation with ammonium molybdate or colorimetric methods using 1,2,4-aminonaphtholsulfonic acid .
- Impurity Profiling : Ion chromatography to detect residual sodium carbonate or disodium phosphate .
Q. What are the standard protocols for preparing this compound buffers in enzymatic assays?
- Buffer Preparation : Dissolve anhydrous NaH2PO4 in deionized water, adjust pH with dibasic sodium phosphate (Na2HPO4) to achieve desired buffering capacity (typically pH 6.0–7.5) .
- Quality Control : Measure ionic strength via conductivity meters and validate using enzymatic activity tests (e.g., NADPH-dependent reductase assays) .
Advanced Research Questions
Q. How can isotopic labeling (e.g., ¹⁸O) in this compound be utilized to study phospho-dynamics in metabolic pathways?
- Methodology : Replace natural ¹⁶O isotopes in NaH2PO4 with stable ¹⁸O to create NaH2P¹⁸O4. Use this in cell culture media for pulse-labeling experiments .
- Analysis : Post-labeling, enrich phosphopeptides via TiO2 affinity chromatography and analyze via LC-MS/MS to track phosphate incorporation in proteins .
- Challenges : Minimize isotopic exchange during sample processing and account for natural ¹⁸O abundance in controls .
Q. What factors influence the stability of this compound under varying experimental conditions?
- Thermal Stability : Phase diagrams indicate hydrate transitions (e.g., NaH2PO4·2H2O → NaH2PO4·H2O at 35°C; anhydrous form above 71°C) .
- Solution Stability : Hydrolysis rates increase at extreme pH (<3 or >9), requiring pH monitoring during long-term storage .
- Storage Recommendations : Anhydrous forms stored in desiccators at 25°C retain >99% purity for 24 months .
Q. How does this compound function as a cofactor in NADP/NADPH-dependent enzymatic systems, and what experimental designs optimize its use?
- Role in Redox Reactions : Acts as a phosphate donor in NADPH synthesis (e.g., glucose-6-phosphate dehydrogenase assays) .
- Optimization Tips :
- Use NADP+ monosodium salt (C21H27N7O17P3·Na) to avoid interference from counterions .
- Pair with magnesium ions to enhance enzymatic binding affinity .
Q. What analytical techniques resolve contradictions in reported solubility data for this compound hydrates?
- Contradictions : Discrepancies arise from differing hydrate forms (monohydrate vs. dihydrate) and temperature-dependent solubility .
- Resolution Strategies :
- Use Extended UNIQUAC models to predict solubility curves .
- Validate experimentally via gravimetric analysis after saturation at controlled temperatures .
Q. Methodological Notes
特性
CAS番号 |
1184-16-3 |
---|---|
分子式 |
C21H27N7NaO17P3 |
分子量 |
765.4 g/mol |
IUPAC名 |
sodium;[[(2R)-5-(6-aminopurin-9-yl)-3-hydroxy-4-phosphonooxyoxolan-2-yl]methoxy-oxidophosphoryl] [(2R,3S,4R)-5-(3-carbamoylpyridin-1-ium-1-yl)-3,4-dihydroxyoxolan-2-yl]methyl phosphate |
InChI |
InChI=1S/C21H28N7O17P3.Na/c22-17-12-19(25-7-24-17)28(8-26-12)21-16(44-46(33,34)35)14(30)11(43-21)6-41-48(38,39)45-47(36,37)40-5-10-13(29)15(31)20(42-10)27-3-1-2-9(4-27)18(23)32;/h1-4,7-8,10-11,13-16,20-21,29-31H,5-6H2,(H7-,22,23,24,25,32,33,34,35,36,37,38,39);/q;+1/p-1/t10-,11-,13-,14?,15-,16?,20?,21?;/m1./s1 |
InChIキー |
JNUMDLCHLVUHFS-HLFIWPCTSA-M |
SMILES |
C1=CC(=C[N+](=C1)C2C(C(C(O2)COP(=O)([O-])OP(=O)([O-])OCC3C(C(C(O3)N4C=NC5=C(N=CN=C54)N)OP(=O)(O)O)O)O)O)C(=O)N.[Na+] |
異性体SMILES |
C1=CC(=C[N+](=C1)C2[C@@H]([C@@H]([C@H](O2)COP(=O)([O-])OP(=O)([O-])OC[C@@H]3C(C(C(O3)N4C=NC5=C(N=CN=C54)N)OP(=O)(O)O)O)O)O)C(=O)N.[Na+] |
正規SMILES |
C1=CC(=C[N+](=C1)C2C(C(C(O2)COP(=O)([O-])OP(=O)([O-])OCC3C(C(C(O3)N4C=NC5=C(N=CN=C54)N)OP(=O)(O)O)O)O)O)C(=O)N.[Na+] |
Key on ui other cas no. |
53-59-8 |
物理的記述 |
White odorless solid; [Bio-Rad Laboratories MSDS] |
ピクトグラム |
Irritant |
関連するCAS |
24292-60-2 |
製品の起源 |
United States |
Retrosynthesis Analysis
AI-Powered Synthesis Planning: Our tool employs the Template_relevance Pistachio, Template_relevance Bkms_metabolic, Template_relevance Pistachio_ringbreaker, Template_relevance Reaxys, Template_relevance Reaxys_biocatalysis model, leveraging a vast database of chemical reactions to predict feasible synthetic routes.
One-Step Synthesis Focus: Specifically designed for one-step synthesis, it provides concise and direct routes for your target compounds, streamlining the synthesis process.
Accurate Predictions: Utilizing the extensive PISTACHIO, BKMS_METABOLIC, PISTACHIO_RINGBREAKER, REAXYS, REAXYS_BIOCATALYSIS database, our tool offers high-accuracy predictions, reflecting the latest in chemical research and data.
Strategy Settings
Precursor scoring | Relevance Heuristic |
---|---|
Min. plausibility | 0.01 |
Model | Template_relevance |
Template Set | Pistachio/Bkms_metabolic/Pistachio_ringbreaker/Reaxys/Reaxys_biocatalysis |
Top-N result to add to graph | 6 |
Feasible Synthetic Routes
試験管内研究製品の免責事項と情報
BenchChemで提示されるすべての記事および製品情報は、情報提供を目的としています。BenchChemで購入可能な製品は、生体外研究のために特別に設計されています。生体外研究は、ラテン語の "in glass" に由来し、生物体の外で行われる実験を指します。これらの製品は医薬品または薬として分類されておらず、FDAから任何の医療状態、病気、または疾患の予防、治療、または治癒のために承認されていません。これらの製品を人間または動物に体内に導入する形態は、法律により厳格に禁止されています。これらのガイドラインに従うことは、研究と実験において法的および倫理的な基準の遵守を確実にするために重要です。