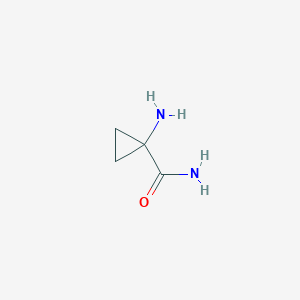
1-アミノシクロプロパン-1-カルボン酸アミド
概要
説明
1-Aminocyclopropane-1-carboxamide is a compound of significant interest in various scientific fields due to its unique structure and properties. It is a derivative of 1-aminocyclopropane-1-carboxylic acid, which is known for its role in the biosynthesis of the plant hormone ethylene . The compound features a cyclopropane ring fused to an amino acid structure, making it a valuable subject for research in organic chemistry and biochemistry.
科学的研究の応用
1-Aminocyclopropane-1-carboxamide has a wide range of applications in scientific research:
Chemistry: It is used as a building block for synthesizing more complex molecules and studying reaction mechanisms.
Biology: The compound plays a role in studying ethylene biosynthesis and signaling pathways in plants.
Industry: It is utilized in the production of plant growth regulators and other agrochemicals.
作用機序
Target of Action
1-Aminocyclopropane-1-carboxamide (ACC) is a non-protein amino acid that acts as the direct precursor of the plant hormone ethylene . It plays a critical role in pollination and seed production by activating proteins similar to those involved in nervous system responses in humans and animals .
Mode of Action
ACC is synthesized from S-adenosyl-L-methionine (SAM) by ACC synthases (ACSs) and subsequently oxidized to ethylene by ACC oxidases (ACOs) . More specifically, ACC signaling promotes secretion of the pollen tube chemoattractant LURE1.2 in ovular sporophytic tissue, thus enhancing pollen tube attraction. Additionally, ACC activates Ca 2+ -containing ion currents via glutamate receptor-like (GLR) channels in root protoplasts .
Biochemical Pathways
The biosynthesis of ACC starts with the conversion of the amino acid methionine to S-adenosyl L-methionine (SAM) by SAM synthetase. SAM is then converted to ACC, which is catalyzed by ACC synthase (ACS). The by-product of this reaction, 5’-methylthioadenosine (MTA), is recycled back into the Yang cycle while ACC is oxidized to ethylene by ACC oxidase (ACO) .
Pharmacokinetics
It is known that acc can be used by soil microorganisms (both bacteria and fungi) as a source of nitrogen and carbon . This suggests that ACC may be metabolized and utilized by various organisms, potentially influencing its bioavailability.
Result of Action
The primary result of ACC’s action is the production of ethylene, a plant hormone that regulates a wide variety of vegetative and developmental processes . Ethylene controls several processes linked to vegetative plant growth but is also a major player in seed germination, fruit ripening, leaf and flower senescence, and abscission .
Action Environment
Environmental factors can influence the action, efficacy, and stability of ACC. For instance, the rate of ACC formation differs in response to developmental, hormonal, and environmental cues . Additionally, using ACC to incubate soils has been proven to induce the gene abundance encoding ACC-deaminases, which may have positive consequences on plant growth and stress tolerance .
生化学分析
Biochemical Properties
1-Aminocyclopropane-1-carboxamide interacts with various enzymes, proteins, and other biomolecules. It is synthesized by the enzyme ACC synthase from methionine and converted to ethylene by ACC oxidase . This process involves the interaction of 1-Aminocyclopropane-1-carboxamide with these enzymes, leading to the production of ethylene .
Cellular Effects
1-Aminocyclopropane-1-carboxamide has significant effects on various types of cells and cellular processes. It influences cell function by acting as a precursor to the plant hormone ethylene . This hormone regulates a wide range of developmental processes and responses to biotic and abiotic stresses .
Molecular Mechanism
The molecular mechanism of action of 1-Aminocyclopropane-1-carboxamide involves its conversion to ethylene. This process is catalyzed by ACC synthase and ACC oxidase . The conversion of 1-Aminocyclopropane-1-carboxamide to ethylene involves binding interactions with these enzymes, leading to changes in gene expression .
Metabolic Pathways
1-Aminocyclopropane-1-carboxamide is involved in the ethylene biosynthesis pathway . This pathway starts with the conversion of the amino acid methionine to S-adenosyl L-methionine (SAM) by SAM synthetase and the subsequent conversion of SAM to 1-Aminocyclopropane-1-carboxamide, which is catalyzed by ACC synthase .
Transport and Distribution
1-Aminocyclopropane-1-carboxamide can be transported throughout the plant over short and long distances
Subcellular Localization
Some studies suggest that ACC oxidase, the enzyme that converts 1-Aminocyclopropane-1-carboxamide to ethylene, is mainly located in the cytosol .
準備方法
The synthesis of 1-aminocyclopropane-1-carboxamide can be achieved through several methods. One common approach involves the alkylation of glycine equivalents with 1,2-electrophiles, followed by intramolecular cyclization of γ-substituted amino acid derivatives . Another method includes the cyclopropanation of alkenes using diazo compounds, ylides, or carbene intermediates . Industrial production methods often focus on optimizing these reactions to achieve high yields and purity.
化学反応の分析
1-Aminocyclopropane-1-carboxamide undergoes various chemical reactions, including:
Oxidation: This reaction can be facilitated by oxidizing agents such as potassium permanganate or hydrogen peroxide, leading to the formation of carboxylic acids.
Reduction: Reduction reactions typically involve reagents like lithium aluminum hydride, resulting in the formation of amines.
The major products formed from these reactions depend on the specific conditions and reagents used.
類似化合物との比較
1-Aminocyclopropane-1-carboxamide can be compared to other similar compounds, such as:
1-Aminocyclopropane-1-carboxylic acid: This compound is the direct precursor to ethylene and shares a similar structure but differs in its functional groups.
1-Aminocyclobutane-1-carboxylic acid: Another analog that has been studied for its effects on plant growth and stress tolerance.
α-Aminoisobutyric acid: Known for its role in enhancing stress tolerance in plants, this compound also shares structural similarities with 1-aminocyclopropane-1-carboxamide.
These comparisons highlight the unique properties and applications of 1-aminocyclopropane-1-carboxamide in various scientific fields.
特性
IUPAC Name |
1-aminocyclopropane-1-carboxamide | |
---|---|---|
Source | PubChem | |
URL | https://pubchem.ncbi.nlm.nih.gov | |
Description | Data deposited in or computed by PubChem | |
InChI |
InChI=1S/C4H8N2O/c5-3(7)4(6)1-2-4/h1-2,6H2,(H2,5,7) | |
Source | PubChem | |
URL | https://pubchem.ncbi.nlm.nih.gov | |
Description | Data deposited in or computed by PubChem | |
InChI Key |
JFGXRDGYMLGBBB-UHFFFAOYSA-N | |
Source | PubChem | |
URL | https://pubchem.ncbi.nlm.nih.gov | |
Description | Data deposited in or computed by PubChem | |
Canonical SMILES |
C1CC1(C(=O)N)N | |
Source | PubChem | |
URL | https://pubchem.ncbi.nlm.nih.gov | |
Description | Data deposited in or computed by PubChem | |
Molecular Formula |
C4H8N2O | |
Source | PubChem | |
URL | https://pubchem.ncbi.nlm.nih.gov | |
Description | Data deposited in or computed by PubChem | |
DSSTOX Substance ID |
DTXSID80470904 | |
Record name | 1-Aminocyclopropane-1-carboxamide | |
Source | EPA DSSTox | |
URL | https://comptox.epa.gov/dashboard/DTXSID80470904 | |
Description | DSSTox provides a high quality public chemistry resource for supporting improved predictive toxicology. | |
Molecular Weight |
100.12 g/mol | |
Source | PubChem | |
URL | https://pubchem.ncbi.nlm.nih.gov | |
Description | Data deposited in or computed by PubChem | |
CAS No. |
137360-55-5 | |
Record name | 1-Aminocyclopropane-1-carboxamide | |
Source | EPA DSSTox | |
URL | https://comptox.epa.gov/dashboard/DTXSID80470904 | |
Description | DSSTox provides a high quality public chemistry resource for supporting improved predictive toxicology. | |
Retrosynthesis Analysis
AI-Powered Synthesis Planning: Our tool employs the Template_relevance Pistachio, Template_relevance Bkms_metabolic, Template_relevance Pistachio_ringbreaker, Template_relevance Reaxys, Template_relevance Reaxys_biocatalysis model, leveraging a vast database of chemical reactions to predict feasible synthetic routes.
One-Step Synthesis Focus: Specifically designed for one-step synthesis, it provides concise and direct routes for your target compounds, streamlining the synthesis process.
Accurate Predictions: Utilizing the extensive PISTACHIO, BKMS_METABOLIC, PISTACHIO_RINGBREAKER, REAXYS, REAXYS_BIOCATALYSIS database, our tool offers high-accuracy predictions, reflecting the latest in chemical research and data.
Strategy Settings
Precursor scoring | Relevance Heuristic |
---|---|
Min. plausibility | 0.01 |
Model | Template_relevance |
Template Set | Pistachio/Bkms_metabolic/Pistachio_ringbreaker/Reaxys/Reaxys_biocatalysis |
Top-N result to add to graph | 6 |
Feasible Synthetic Routes
試験管内研究製品の免責事項と情報
BenchChemで提示されるすべての記事および製品情報は、情報提供を目的としています。BenchChemで購入可能な製品は、生体外研究のために特別に設計されています。生体外研究は、ラテン語の "in glass" に由来し、生物体の外で行われる実験を指します。これらの製品は医薬品または薬として分類されておらず、FDAから任何の医療状態、病気、または疾患の予防、治療、または治癒のために承認されていません。これらの製品を人間または動物に体内に導入する形態は、法律により厳格に禁止されています。これらのガイドラインに従うことは、研究と実験において法的および倫理的な基準の遵守を確実にするために重要です。