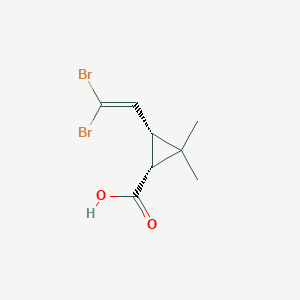
デルタメトリン酸
概要
説明
Deltamethrinic acid is a synthetic compound inspired by the natural compounds found in the flower head of the plant Chrysanthemum cinerariifolium. It is a key intermediate in the synthesis of deltamethrin, a highly effective insecticide with low toxicity to mammals .
科学的研究の応用
Agricultural Applications
Deltamethrinic acid is primarily utilized in agriculture as an effective insecticide. Its efficacy against a broad spectrum of pests makes it a valuable tool for pest management strategies.
- Insecticidal Efficacy : Deltamethrinic acid exhibits high insecticidal activity against various agricultural pests, including aphids, beetles, and caterpillars. The compound's effectiveness is attributed to its ability to disrupt the nervous system of insects by binding to sodium channels, leading to paralysis and death.
- Chiral Isomers : Research indicates that the cis isomer of deltamethrinic acid shows significantly greater insecticidal activity compared to its trans counterpart. The enantiomerically pure form of deltamethrin has been shown to provide similar efficacy with reduced environmental impact, highlighting the importance of chiral selectivity in pesticide formulation .
Environmental Impact and Safety
The environmental safety profile of deltamethrinic acid is a critical consideration in its application.
- Reduced Toxicity : Compared to other pesticides, deltamethrinic acid has lower toxicity levels for non-target organisms, including mammals and beneficial insects. This characteristic makes it a preferred choice in integrated pest management (IPM) systems aimed at minimizing ecological disruption .
- Degradation Studies : Recent studies have focused on the degradation pathways of deltamethrin in various environments. Understanding these pathways is essential for assessing the long-term impact of deltamethrinic acid on ecosystems. For instance, research utilizing NMR spectroscopy has identified degradation products that may influence both efficacy and safety profiles .
Chemical Research Applications
Deltamethrinic acid also serves as a significant compound in chemical research.
- Chiral Separations : The unique structure of deltamethrinic acid allows for its use in chiral separation studies. Cyclodextrin-based methods have been employed to achieve effective separations of its isomers, facilitating the synthesis of enantiomerically pure compounds with desired biological activities .
- Mechanistic Studies : Investigations into the mechanistic action of deltamethrinic acid have revealed insights into its neurotoxic potential. Studies using in vitro models have demonstrated binding affinities to sodium channels and ryanodine receptors at low concentrations, which are critical for understanding developmental neurotoxicity risks associated with pesticide exposure .
Case Study 1: Insecticide Efficacy
A study evaluating the insecticidal effectiveness of different formulations containing deltamethrinic acid found that formulations with higher concentrations of the cis isomer resulted in significantly lower pest populations compared to those with mixed isomers. This underscores the importance of selecting appropriate formulations for effective pest control.
Case Study 2: Environmental Safety Assessment
A comprehensive risk assessment conducted by the European Food Safety Authority (EFSA) examined the developmental neurotoxicity (DNT) potential of deltamethrin. The assessment integrated data from multiple studies, concluding that while deltamethrin poses some neurotoxic risks at high exposure levels, its application under regulated conditions minimizes these risks significantly .
Data Tables
作用機序
Target of Action
Deltamethrinic acid is a synthetic compound inspired by the structures present in the flower head of the plant Chrysanthemum cinerariifolium . It is a pyrethroid ester insecticide that plays a key role in controlling malaria vectors . The primary targets of deltamethrinic acid are pests and parasites, particularly those that pose a threat to agriculture and public health .
Mode of Action
Deltamethrinic acid interacts with its targets by disrupting their nervous system . It binds to the voltage-gated sodium channels in the nerve cells of the pests, causing a delay in the closure of these channels. This leads to an influx of sodium ions, resulting in nerve cell depolarization and eventual paralysis of the pest .
Biochemical Pathways
It is known that deltamethrinic acid interferes with the normal functioning of the nervous system of pests, leading to their paralysis and death
Pharmacokinetics
The pharmacokinetics of deltamethrinic acid involves its absorption, distribution, metabolism, and excretion (ADME). It is absorbed in the gastrointestinal (GI) tract as a saturable process mediated by phase III efflux transporters, which pump deltamethrinic acid out of the intestinal enterocytes into the GI tract lumen . This results in minimal net absorption at low concentrations and increasing absorption at higher concentrations .
Result of Action
The molecular and cellular effects of deltamethrinic acid’s action primarily involve the disruption of the nervous system of pests, leading to their paralysis and death . Chronic exposure to deltamethrinic acid has been associated with various adverse health effects, including neurotoxicity, reproductive toxicity, and carcinogenicity .
Action Environment
Environmental factors can influence the action, efficacy, and stability of deltamethrinic acid. It is highly toxic to a wide range of non-target organisms, including mammals, birds, fish, and bees . Mammals and birds can be exposed to deltamethrinic acid through contaminated food, water, or direct contact with the pesticide . Therefore, the use of deltamethrinic acid needs to be regulated and monitored to ensure its safe use and protect the environment and human health .
生化学分析
Biochemical Properties
Deltamethrinic acid belongs to the pyrethroids family, a class of insecticides used to control a wide range of arthropods. It is a broad-spectrum insecticide, meaning it is effective against a wide range of insect species
Cellular Effects
Deltamethrin, the ester of deltamethrinic acid, is known to be neurotoxic and can disrupt the nervous system . It is generally considered safe to use around humans, but it is still toxic to aquatic life, particularly fish
Molecular Mechanism
Deltamethrin, the ester of deltamethrinic acid, works by opening up presynaptic sodium channels, interfering with the transport between sodium and potassium cations, thereby disrupting the entire nervous system
Temporal Effects in Laboratory Settings
It is known that deltamethrin, the ester of deltamethrinic acid, is highly effective as an insecticide .
Dosage Effects in Animal Models
It is known that deltamethrin, the ester of deltamethrinic acid, is toxic to aquatic life, particularly fish .
Metabolic Pathways
It is known that deltamethrin, the ester of deltamethrinic acid, can be degraded by certain bacterial co-cultures .
Transport and Distribution
It is known that deltamethrin, the ester of deltamethrinic acid, can be introduced into the food chain through bioaccumulation in Pacific oysters .
Subcellular Localization
It is known that deltamethrin, the ester of deltamethrinic acid, can disrupt the nervous system .
準備方法
Synthetic Routes and Reaction Conditions
Deltamethrinic acid can be synthesized through various synthetic routes. One common method involves the reaction of 3-(2,2-dibromoethenyl)-2,2-dimethylcyclopropanecarboxylic acid with appropriate reagents under controlled conditions . The reaction typically requires the use of catalysts and specific temperature and pressure conditions to ensure high yield and purity.
Industrial Production Methods
Industrial production of deltamethrinic acid involves large-scale synthesis using optimized reaction conditions to maximize efficiency and minimize costs. The process often includes steps such as purification and crystallization to obtain the final product in a highly pure form .
化学反応の分析
Types of Reactions
Deltamethrinic acid undergoes various chemical reactions, including:
Oxidation: It can be oxidized to form corresponding oxides.
Reduction: Reduction reactions can convert it into different reduced forms.
Substitution: It can undergo substitution reactions where one or more atoms are replaced by other atoms or groups.
Common Reagents and Conditions
Common reagents used in these reactions include oxidizing agents like potassium permanganate, reducing agents like lithium aluminum hydride, and various catalysts to facilitate substitution reactions. The conditions for these reactions vary depending on the desired product and include specific temperatures, pressures, and solvents .
Major Products Formed
The major products formed from these reactions depend on the type of reaction and the reagents used. For example, oxidation of deltamethrinic acid can produce oxides, while substitution reactions can yield various substituted derivatives .
類似化合物との比較
Similar Compounds
Compounds similar to deltamethrinic acid include other pyrethroid acids such as permethrinic acid and cypermethrinic acid. These compounds share similar structures and insecticidal properties but differ in their specific chemical configurations and activities .
Uniqueness
Deltamethrinic acid is unique due to its high insecticidal activity and low mammalian toxicity. Its structure, inspired by natural compounds, allows it to effectively target insect nervous systems while being safe for use around humans and animals .
生物活性
Deltamethrin, a synthetic pyrethroid insecticide, is widely used in agriculture and public health for pest control. Its active metabolite, deltamethrinic acid, exhibits significant biological activity that has been the subject of various studies. This article explores the biological effects of deltamethrinic acid, focusing on its neurotoxic effects, oxidative stress induction, and implications for human health.
Deltamethrinic acid primarily acts on the nervous system by modifying sodium channel activity in neurons. This leads to prolonged depolarization and increased neurotransmitter release, particularly affecting the dopaminergic system. Research indicates that exposure to deltamethrin can disrupt dopamine receptor expression and alter locomotor activity in animal models, such as zebrafish. Notably, developmental exposure to deltamethrin has been shown to cause persistent changes in dopaminergic gene expression and neurochemistry, leading to locomotor deficits .
Neurotoxic Effects
A study on zebrafish demonstrated that developmental exposure to low doses of deltamethrin resulted in significant alterations in locomotor behavior. Specifically, larval zebrafish exposed to deltamethrin exhibited increased swim activity associated with decreased expression of dopamine receptors (D1 and D2) and increased levels of homovanillic acid, a dopamine metabolite . This suggests that deltamethrin disrupts normal dopaminergic signaling pathways.
Table 1: Summary of Neurotoxic Effects of Deltamethrinic Acid
Oxidative Stress Induction
Deltamethrinic acid is also known to induce oxidative stress in various biological systems. A study conducted on male rats revealed that short-term oral exposure to deltamethrin significantly increased lipid peroxidation markers while decreasing antioxidant enzyme activities such as superoxide dismutase (SOD) and glutathione S-transferase (GST) . The presence of Vitamin E was found to mitigate some of these adverse effects, indicating a potential protective role against oxidative damage caused by deltamethrin.
Table 2: Biochemical Alterations Induced by Deltamethrinic Acid
Parameter | Control Group | Deltamethrin Group | Vitamin E + Deltamethrin Group |
---|---|---|---|
TBARS (Lipid Peroxidation) | Baseline | Significantly ↑ | ↓ |
SOD Activity | Baseline | Significantly ↓ | ↑ |
AST Activity | Baseline | Significantly ↑ | ↓ |
Total Lipids | Baseline | Significantly ↑ | ↓ |
Case Studies on Human Exposure
Recent case studies have highlighted the clinical implications of deltamethrin exposure in humans. One case involved a patient who ingested deltamethrin in an attempt at self-harm, presenting with symptoms similar to organophosphate poisoning, including fasciculations and altered mental status . This case underscores the need for awareness regarding the potential toxicity of deltamethrin and its ability to mimic other toxic compounds.
Table 3: Clinical Features Observed in Deltamethrin Poisoning
Symptom | Description |
---|---|
Fasciculations | Muscle twitches observed |
Respiratory Distress | Tachypnea noted |
Neurological Symptoms | Myoclonic seizures; altered consciousness |
Positive Atropine Challenge | Mimics organophosphate poisoning signs |
特性
IUPAC Name |
(1R,3R)-3-(2,2-dibromoethenyl)-2,2-dimethylcyclopropane-1-carboxylic acid | |
---|---|---|
Source | PubChem | |
URL | https://pubchem.ncbi.nlm.nih.gov | |
Description | Data deposited in or computed by PubChem | |
InChI |
InChI=1S/C8H10Br2O2/c1-8(2)4(3-5(9)10)6(8)7(11)12/h3-4,6H,1-2H3,(H,11,12)/t4-,6-/m0/s1 | |
Source | PubChem | |
URL | https://pubchem.ncbi.nlm.nih.gov | |
Description | Data deposited in or computed by PubChem | |
InChI Key |
MDIQXIJPQWLFSD-NJGYIYPDSA-N | |
Source | PubChem | |
URL | https://pubchem.ncbi.nlm.nih.gov | |
Description | Data deposited in or computed by PubChem | |
Canonical SMILES |
CC1(C(C1C(=O)O)C=C(Br)Br)C | |
Source | PubChem | |
URL | https://pubchem.ncbi.nlm.nih.gov | |
Description | Data deposited in or computed by PubChem | |
Isomeric SMILES |
CC1([C@H]([C@H]1C(=O)O)C=C(Br)Br)C | |
Source | PubChem | |
URL | https://pubchem.ncbi.nlm.nih.gov | |
Description | Data deposited in or computed by PubChem | |
Molecular Formula |
C8H10Br2O2 | |
Source | PubChem | |
URL | https://pubchem.ncbi.nlm.nih.gov | |
Description | Data deposited in or computed by PubChem | |
DSSTOX Substance ID |
DTXSID40873159 | |
Record name | (1R-cis)-Decamethrinic acid | |
Source | EPA DSSTox | |
URL | https://comptox.epa.gov/dashboard/DTXSID40873159 | |
Description | DSSTox provides a high quality public chemistry resource for supporting improved predictive toxicology. | |
Molecular Weight |
297.97 g/mol | |
Source | PubChem | |
URL | https://pubchem.ncbi.nlm.nih.gov | |
Description | Data deposited in or computed by PubChem | |
CAS No. |
53179-78-5, 63597-73-9 | |
Record name | Deltamethrinic acid | |
Source | ChemIDplus | |
URL | https://pubchem.ncbi.nlm.nih.gov/substance/?source=chemidplus&sourceid=0053179785 | |
Description | ChemIDplus is a free, web search system that provides access to the structure and nomenclature authority files used for the identification of chemical substances cited in National Library of Medicine (NLM) databases, including the TOXNET system. | |
Record name | Cyclopropanecarboxylic acid, 3-(2,2-dibromoethenyl)-2,2-dimethyl-, cis- | |
Source | ChemIDplus | |
URL | https://pubchem.ncbi.nlm.nih.gov/substance/?source=chemidplus&sourceid=0063597739 | |
Description | ChemIDplus is a free, web search system that provides access to the structure and nomenclature authority files used for the identification of chemical substances cited in National Library of Medicine (NLM) databases, including the TOXNET system. | |
Record name | (1R-cis)-Decamethrinic acid | |
Source | EPA DSSTox | |
URL | https://comptox.epa.gov/dashboard/DTXSID40873159 | |
Description | DSSTox provides a high quality public chemistry resource for supporting improved predictive toxicology. | |
Record name | (1R-cis)-3-(2,2-dibromoethenyl)-2,2-dimethylcyclopropane carboxylic acid | |
Source | European Chemicals Agency (ECHA) | |
URL | https://echa.europa.eu/information-on-chemicals | |
Description | The European Chemicals Agency (ECHA) is an agency of the European Union which is the driving force among regulatory authorities in implementing the EU's groundbreaking chemicals legislation for the benefit of human health and the environment as well as for innovation and competitiveness. | |
Explanation | Use of the information, documents and data from the ECHA website is subject to the terms and conditions of this Legal Notice, and subject to other binding limitations provided for under applicable law, the information, documents and data made available on the ECHA website may be reproduced, distributed and/or used, totally or in part, for non-commercial purposes provided that ECHA is acknowledged as the source: "Source: European Chemicals Agency, http://echa.europa.eu/". Such acknowledgement must be included in each copy of the material. ECHA permits and encourages organisations and individuals to create links to the ECHA website under the following cumulative conditions: Links can only be made to webpages that provide a link to the Legal Notice page. | |
Record name | DELTAMETHRINIC ACID | |
Source | FDA Global Substance Registration System (GSRS) | |
URL | https://gsrs.ncats.nih.gov/ginas/app/beta/substances/XHE31R77PQ | |
Description | The FDA Global Substance Registration System (GSRS) enables the efficient and accurate exchange of information on what substances are in regulated products. Instead of relying on names, which vary across regulatory domains, countries, and regions, the GSRS knowledge base makes it possible for substances to be defined by standardized, scientific descriptions. | |
Explanation | Unless otherwise noted, the contents of the FDA website (www.fda.gov), both text and graphics, are not copyrighted. They are in the public domain and may be republished, reprinted and otherwise used freely by anyone without the need to obtain permission from FDA. Credit to the U.S. Food and Drug Administration as the source is appreciated but not required. | |
Synthesis routes and methods
Procedure details
Retrosynthesis Analysis
AI-Powered Synthesis Planning: Our tool employs the Template_relevance Pistachio, Template_relevance Bkms_metabolic, Template_relevance Pistachio_ringbreaker, Template_relevance Reaxys, Template_relevance Reaxys_biocatalysis model, leveraging a vast database of chemical reactions to predict feasible synthetic routes.
One-Step Synthesis Focus: Specifically designed for one-step synthesis, it provides concise and direct routes for your target compounds, streamlining the synthesis process.
Accurate Predictions: Utilizing the extensive PISTACHIO, BKMS_METABOLIC, PISTACHIO_RINGBREAKER, REAXYS, REAXYS_BIOCATALYSIS database, our tool offers high-accuracy predictions, reflecting the latest in chemical research and data.
Strategy Settings
Precursor scoring | Relevance Heuristic |
---|---|
Min. plausibility | 0.01 |
Model | Template_relevance |
Template Set | Pistachio/Bkms_metabolic/Pistachio_ringbreaker/Reaxys/Reaxys_biocatalysis |
Top-N result to add to graph | 6 |
Feasible Synthetic Routes
Q1: What makes deltamethrinic acid a potent insecticide?
A1: Deltamethrinic acid, like other pyrethroids, exerts its insecticidal effect by targeting the nervous system of insects. [] Specifically, it acts on voltage-gated sodium channels, preventing their closure and leading to hyper-excitation of nerve cells. This ultimately results in paralysis and death of the insect. []
Q2: How does the structure of deltamethrinic acid contribute to its activity?
A2: Deltamethrinic acid possesses both geometric and chiral isomerism, resulting in several possible stereoisomers. [] The (1R)-cis isomer exhibits the most potent insecticidal activity. Research has shown that the cis isomers generally demonstrate higher selectivity for the insect sodium channels compared to the trans isomers. [] This highlights the crucial role of stereochemistry in the biological activity of deltamethrinic acid.
Q3: Can you elaborate on the synthesis of deltamethrinic acid and its challenges?
A3: Several synthetic routes have been developed for deltamethrinic acid, many focusing on achieving enantiomeric purity of the active (1R)-cis isomer. One approach utilizes a chemo-enzymatic process starting from a racemic mixture. [] This method employs a lipase enzyme to selectively resolve the desired enantiomer, offering a sustainable alternative to traditional chemical synthesis. Another approach utilizes chiral bicyclic lactams as intermediates in a diastereoselective cyclopropanation reaction, leading to enantiomerically pure deltamethrinic acid. [, , , , ] These studies highlight the ongoing efforts in optimizing deltamethrinic acid synthesis for improved efficiency and stereochemical control.
Q4: How stable is deltamethrinic acid under different environmental conditions?
A4: Research on a closely related compound, cis-permethrin, provides insight into the stability of deltamethrinic acid. Studies have shown that the presence of certain salts, particularly potassium chlorate, can significantly impact the thermal stability of these pyrethroids, leading to decomposition and isomerization at high temperatures. [] This information is crucial for understanding the degradation pathways of deltamethrinic acid and its potential environmental impact.
Q5: What analytical techniques are employed to study deltamethrinic acid?
A5: Various analytical methods are employed to characterize and quantify deltamethrinic acid. High-performance liquid chromatography (HPLC) is widely used for separating and analyzing different isomers of pyrethroids, including deltamethrinic acid. [] This technique, often coupled with UV detection, allows for the determination of enantiomeric purity and quantification of deltamethrinic acid in various matrices.
試験管内研究製品の免責事項と情報
BenchChemで提示されるすべての記事および製品情報は、情報提供を目的としています。BenchChemで購入可能な製品は、生体外研究のために特別に設計されています。生体外研究は、ラテン語の "in glass" に由来し、生物体の外で行われる実験を指します。これらの製品は医薬品または薬として分類されておらず、FDAから任何の医療状態、病気、または疾患の予防、治療、または治癒のために承認されていません。これらの製品を人間または動物に体内に導入する形態は、法律により厳格に禁止されています。これらのガイドラインに従うことは、研究と実験において法的および倫理的な基準の遵守を確実にするために重要です。