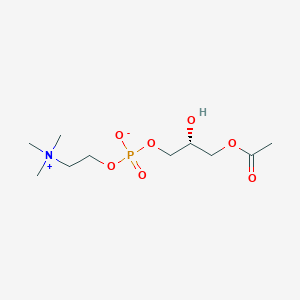
Lysophosphatidylcholine
概要
説明
Lysophosphatidylcholines (LPC, lysoPC), also known as lysolecithins, are a class of chemical compounds derived from phosphatidylcholines . They are produced within cells mainly by the enzyme phospholipase A2, which removes one of the fatty acid groups from phosphatidylcholine to produce LPC . They are present as minor phospholipids in the cell membrane (≤ 3%) and in the blood plasma (8–12%) .
Synthesis Analysis
LPCs are produced within cells mainly by the enzyme phospholipase A2, which removes one of the fatty acid groups from phosphatidylcholine to produce LPC . They can also be produced through ethanolysis of phosphatidylcholine (PC) in n-hexane or solvent-free media catalyzed by Novozym 435 (from Candida antarctica), Lipozyme TLIM (from Thermomcyces lanuginosus) and Lipozyme RMIM (from Rhizomucor miehei) .Molecular Structure Analysis
The general chemical structure of lysophosphatidylcholines consists of a glycerol backbone linked to a fatty acid and a phosphocholine molecule . The fatty acid can be of variable length, hydroxylated, and contain double bonds .Chemical Reactions Analysis
LPCs are quickly metabolized by lysophospholipase and LPC-acyltransferase, they last only shortly in vivo . By replacing the acyl-group within the LPC with an alkyl-group, alkyl-lysophospholipids (ALP) were synthesized .Physical And Chemical Properties Analysis
LPCs are a hydrolyzed phospholipid that is generated from the action of either phospholipase PLA 1 or PLA 2 . There are two types of LPC; 1-LPC (where the omega-3 fatty acid at the sn -2 position is acylated) and 2-LPC (where the omega-3 fatty acid at the sn -1 position is acylated) .科学的研究の応用
Cardiovascular Health
LPC is recognized as a key marker associated with cardiovascular diseases. It contributes to vascular inflammation by increasing the expression of adhesion molecules and the production of chemokines from endothelial cells .
Neurodegenerative Diseases
Studies have linked LPC to neurodegenerative diseases, suggesting its role as a biomarker and potential target for therapeutic intervention .
Lipid Metabolism
LPC metabolism is complex and involves an enzymatic cascade that has been the focus of recent clinical lipidomic studies .
Omega-3 Fatty Acid Bioavailability
LPC-omega-3, derived from marine fish, is considered important for brain health due to its high bioavailability of omega-3 fatty acids .
Autotaxin Pathway
Research has begun to focus on LPC as a potential target for therapeutic intervention through the autotaxin pathway, which yields lysophosphatidic acid (LPA) and choline .
Lysophosphatidylcholine Acyltransferase (LPCAT) Pathway
The reacylation pathway to form phosphatidylcholine (PC) is catalyzed by LPCAT, which has been a subject of research for accurate analysis of individual LPC species .
作用機序
Lysophosphatidylcholine (LPC), also known as lysolecithins, is a class of lipid biomolecule that plays a significant role in various biological processes . This article provides an overview of the mechanism of action of LPC, focusing on its targets, mode of action, biochemical pathways, pharmacokinetics, results of action, and the influence of environmental factors on its action.
Target of Action
LPC exerts its effects through several targets, including G protein-coupled signaling receptors, Toll-like receptors, and ion channels . These targets mediate the pleiotropic effects of LPC, which is increasingly recognized as a key factor in pathological states, particularly inflammation and atherosclerosis development .
Mode of Action
LPC interacts with its targets to activate several second messengers . For instance, in human monocytes, LPC has been found to mobilize calcium (Ca2+). This suggests that the action mechanism of LPC may involve Ca2+ influx across the plasma membrane .
Biochemical Pathways
LPC is primarily derived from the cleavage of phosphatidylcholine (PC) by phospholipase A2 (PLA2) . It can be catabolized to other substances through different enzymatic pathways . A hydrolytic pathway, catalyzed by autotaxin (ATX), yields lysophosphatidic acid (LPA) and choline. A reacylation pathway, catalyzed by lysophosphatidylcholine acyltransferase (LPCAT), forms PC .
Pharmacokinetics
The pharmacokinetics of LPC is complex and involves several enzymes that coordinate to maintain LPC homeostasis . Overexpression or enhanced activity of PLA2 increases the LPC content in modified low-density lipoprotein (LDL) and oxidized LDL, which play significant roles in the development of atherosclerotic plaques and endothelial dysfunction . The intracellular enzyme LPCAT cannot directly remove LPC from circulation .
Result of Action
LPC has been associated with various pathological conditions. For instance, it has been linked to the development of chronic pain . The injury of nervous tissues promotes oxidative stress and lipid peroxidation, as well as excessive accumulation of LPC, enhancing the membrane hyperexcitability to induce chronic pain . Moreover, LPC induces monocyte chemoattractant protein-1 and inflammatory cytokine expression and damages endothelial cells .
Action Environment
The action of LPC can be influenced by various environmental factors. For instance, the presence of Acyl-CoA is necessary for the conversion of LPC to PC by LPCAT . Furthermore, the activity of PLA2, which is involved in the formation of LPC, can be enhanced under certain conditions, leading to increased LPC content .
Safety and Hazards
将来の方向性
特性
IUPAC Name |
[(2R)-3-acetyloxy-2-hydroxypropyl] 2-(trimethylazaniumyl)ethyl phosphate | |
---|---|---|
Source | PubChem | |
URL | https://pubchem.ncbi.nlm.nih.gov | |
Description | Data deposited in or computed by PubChem | |
InChI |
InChI=1S/C10H22NO7P/c1-9(12)16-7-10(13)8-18-19(14,15)17-6-5-11(2,3)4/h10,13H,5-8H2,1-4H3/t10-/m1/s1 | |
Source | PubChem | |
URL | https://pubchem.ncbi.nlm.nih.gov | |
Description | Data deposited in or computed by PubChem | |
InChI Key |
RYCNUMLMNKHWPZ-SNVBAGLBSA-N | |
Source | PubChem | |
URL | https://pubchem.ncbi.nlm.nih.gov | |
Description | Data deposited in or computed by PubChem | |
Canonical SMILES |
CC(=O)OCC(COP(=O)([O-])OCC[N+](C)(C)C)O | |
Source | PubChem | |
URL | https://pubchem.ncbi.nlm.nih.gov | |
Description | Data deposited in or computed by PubChem | |
Isomeric SMILES |
CC(=O)OC[C@H](COP(=O)([O-])OCC[N+](C)(C)C)O | |
Source | PubChem | |
URL | https://pubchem.ncbi.nlm.nih.gov | |
Description | Data deposited in or computed by PubChem | |
Molecular Formula |
C10H22NO7P | |
Source | PubChem | |
URL | https://pubchem.ncbi.nlm.nih.gov | |
Description | Data deposited in or computed by PubChem | |
Molecular Weight |
299.26 g/mol | |
Source | PubChem | |
URL | https://pubchem.ncbi.nlm.nih.gov | |
Description | Data deposited in or computed by PubChem | |
Product Name |
Lysophosphatidylcholine | |
CAS RN |
9008-30-4 | |
Record name | Lysophosphatidylcholine, soybean | |
Source | ChemIDplus | |
URL | https://pubchem.ncbi.nlm.nih.gov/substance/?source=chemidplus&sourceid=0009008304 | |
Description | ChemIDplus is a free, web search system that provides access to the structure and nomenclature authority files used for the identification of chemical substances cited in National Library of Medicine (NLM) databases, including the TOXNET system. | |
Record name | Lysophosphatidylcholines | |
Source | European Chemicals Agency (ECHA) | |
URL | https://echa.europa.eu/substance-information/-/substanceinfo/100.029.728 | |
Description | The European Chemicals Agency (ECHA) is an agency of the European Union which is the driving force among regulatory authorities in implementing the EU's groundbreaking chemicals legislation for the benefit of human health and the environment as well as for innovation and competitiveness. | |
Explanation | Use of the information, documents and data from the ECHA website is subject to the terms and conditions of this Legal Notice, and subject to other binding limitations provided for under applicable law, the information, documents and data made available on the ECHA website may be reproduced, distributed and/or used, totally or in part, for non-commercial purposes provided that ECHA is acknowledged as the source: "Source: European Chemicals Agency, http://echa.europa.eu/". Such acknowledgement must be included in each copy of the material. ECHA permits and encourages organisations and individuals to create links to the ECHA website under the following cumulative conditions: Links can only be made to webpages that provide a link to the Legal Notice page. | |
Retrosynthesis Analysis
AI-Powered Synthesis Planning: Our tool employs the Template_relevance Pistachio, Template_relevance Bkms_metabolic, Template_relevance Pistachio_ringbreaker, Template_relevance Reaxys, Template_relevance Reaxys_biocatalysis model, leveraging a vast database of chemical reactions to predict feasible synthetic routes.
One-Step Synthesis Focus: Specifically designed for one-step synthesis, it provides concise and direct routes for your target compounds, streamlining the synthesis process.
Accurate Predictions: Utilizing the extensive PISTACHIO, BKMS_METABOLIC, PISTACHIO_RINGBREAKER, REAXYS, REAXYS_BIOCATALYSIS database, our tool offers high-accuracy predictions, reflecting the latest in chemical research and data.
Strategy Settings
Precursor scoring | Relevance Heuristic |
---|---|
Min. plausibility | 0.01 |
Model | Template_relevance |
Template Set | Pistachio/Bkms_metabolic/Pistachio_ringbreaker/Reaxys/Reaxys_biocatalysis |
Top-N result to add to graph | 6 |
Feasible Synthetic Routes
試験管内研究製品の免責事項と情報
BenchChemで提示されるすべての記事および製品情報は、情報提供を目的としています。BenchChemで購入可能な製品は、生体外研究のために特別に設計されています。生体外研究は、ラテン語の "in glass" に由来し、生物体の外で行われる実験を指します。これらの製品は医薬品または薬として分類されておらず、FDAから任何の医療状態、病気、または疾患の予防、治療、または治癒のために承認されていません。これらの製品を人間または動物に体内に導入する形態は、法律により厳格に禁止されています。これらのガイドラインに従うことは、研究と実験において法的および倫理的な基準の遵守を確実にするために重要です。