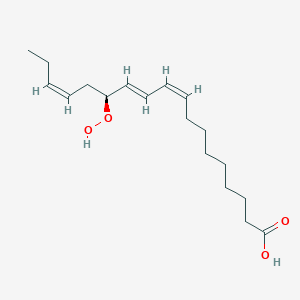
(9Z,11E,13S,15Z)-13-hydroperoxyoctadeca-9,11,15-trienoic acid
説明
“(9Z,11E,13S,15Z)-13-Hydroxyoctadeca-9,11,15-trienoic acid” belongs to the class of organic compounds known as lineolic acids and derivatives . These are derivatives of lineolic acid, a polyunsaturated omega-6 18 carbon long fatty acid, with two CC double bonds .
Synthesis Analysis
This compound is a lipid hydroperoxide, obtained by the formal substitution of a hydrogen at position 9 of (9Z,11E,15Z)-octadeca-9,11,15-trienoic acid by a hydroperoxy group (the 13S-stereoisomer) . Biochemically, it is an 18-C rearrangement and primary peroxidation product of α-linolenic acid, an intermediate in prostaglandin and leukotriene metabolism, and the substrate of the lyase enzyme hydroperoxide dehydratase .Molecular Structure Analysis
The molecular formula of this compound is C18H30O4 . Its average mass is 310.42840 and its mono-isotopic mass is 310.21441 . The InChI string representation of its structure isInChI=1S/C18H30O4/c1- 2- 3- 11- 14- 17 (22- 21) 15- 12- 9- 7- 5- 4- 6- 8- 10- 13- 16- 18 (19) 20/h3,7,9,11- 12,15,17,21H,2,4- 6,8,10,13- 14,16H2,1H3, (H,19,20) /b9- 7- ,11- 3- ,15- 12+/t17- /m0/s1
. Chemical Reactions Analysis
“(9Z,11E,13S,15Z)-13-hydroperoxyoctadeca-9,11,15-trienoate” is a hydroperoxyoctadecatrienoate . It is a conjugate base of "(9Z,11E,13S,15Z)-13-hydroperoxyoctadeca-9,11,15-trienoic acid" .Physical And Chemical Properties Analysis
The compound has a boiling point of 454.5±40.0 °C . Its density is 1.016±0.06 g/cm3 . It has 4 hydrogen bond acceptors and 2 hydrogen bond donors . It has 14 freely rotating bonds . Its polar surface area is 70 Å2 .科学的研究の応用
Role in Lipid Metabolic Disorder and Oxidative Stress
13(S)-Hydroperoxylinolenic acid plays a significant role in lipid metabolic disorder and oxidative stress, particularly in the liver of freshwater drum, Aplodinotus grunniens . High-fat-diet-induced lipid deposition was associated with increased condition factor (CF), viscerosomatic index (VSI), and hepatosomatic index (HSI). Lipid deposition led to physiological and metabolic disorders, inhibited antioxidant capacity, and exacerbated the burden of lipid metabolism .
Involvement in Lipid Metabolism
This compound is involved in lipid metabolism. Lipid deposition promoted fatty acid synthesis but suppressed catabolism. The transcriptome and metabolome showed significant enrichment of lipid metabolism and antioxidant pathways .
Interaction with Peroxisome Proliferator-Activated Receptor (PPAR)
The interaction analysis suggested that peroxisome proliferator-activated receptor (PPAR)-mediated 13-S-hydroxyoctadecenoic acid (13 (s)-HODE) could serve as the key target in regulating lipid metabolism and oxidative stress during lipid deposition in A. grunniens .
Role in Lipid Uptake, Cholesterol Transport, and Apoptosis
Studies have shown that 13 (s)-HODE can increase lipid uptake, return cholesterol transport, and apoptosis. As a marker of oxidative stress, high-fat diets also lead to increased 13 (s)-HODE .
Potential Role in Lipid Expenditure and Physiological Homeostasis
With a lipid intake restriction experiment, PPARs were confirmed to regulate lipid expenditure and physiological homeostasis in A. grunniens .
作用機序
Target of Action
13(S)-Hydroperoxylinolenic acid, also known as 13(S)-HpOTrE, primarily targets the Peroxisome Proliferator-Activated Receptor (PPAR) . PPARs are a group of nuclear receptor proteins that function as transcription factors regulating the expression of genes. They play essential roles in the regulation of cellular differentiation, development, metabolism, and tumorigenesis .
Mode of Action
The interaction of 13(S)-HpOTrE with PPARs leads to significant changes in cellular functions. Specifically, 13(S)-HpOTrE activates the transcription factor PPARγ . This activation stimulates the production of two receptors on the surface of macrophages: CD36, a scavenger receptor for oxidized low-density lipoproteins, native lipoproteins, oxidized phospholipids, and long-chain fatty acids; and adipocyte protein 2 (aP2), a fatty acid-binding protein . This interaction may cause macrophages to increase their uptake of these lipids, transitioning to lipid-laden foam cells .
Biochemical Pathways
The activation of PPARs by 13(S)-HpOTrE affects several biochemical pathways. The transcriptome and metabolome show significant enrichment of lipid metabolism and antioxidant pathways . This compound promotes fatty acid synthesis but suppresses catabolism . It is involved in the regulation of lipid metabolism and oxidative stress during lipid deposition .
Result of Action
The activation of PPARs by 13(S)-HpOTrE leads to increased lipid uptake by macrophages, which can transition into lipid-laden foam cells . This process contributes to plaque formation . Additionally, the compound is involved in lipid metabolic disorder and oxidative stress in the liver of freshwater drum, Aplodinotus grunniens .
特性
IUPAC Name |
(9Z,11E,13S,15Z)-13-hydroperoxyoctadeca-9,11,15-trienoic acid | |
---|---|---|
Source | PubChem | |
URL | https://pubchem.ncbi.nlm.nih.gov | |
Description | Data deposited in or computed by PubChem | |
InChI |
InChI=1S/C18H30O4/c1-2-3-11-14-17(22-21)15-12-9-7-5-4-6-8-10-13-16-18(19)20/h3,7,9,11-12,15,17,21H,2,4-6,8,10,13-14,16H2,1H3,(H,19,20)/b9-7-,11-3-,15-12+/t17-/m0/s1 | |
Source | PubChem | |
URL | https://pubchem.ncbi.nlm.nih.gov | |
Description | Data deposited in or computed by PubChem | |
InChI Key |
UYQGVDXDXBAABN-FQSPHKRJSA-N | |
Source | PubChem | |
URL | https://pubchem.ncbi.nlm.nih.gov | |
Description | Data deposited in or computed by PubChem | |
Canonical SMILES |
CCC=CCC(C=CC=CCCCCCCCC(=O)O)OO | |
Source | PubChem | |
URL | https://pubchem.ncbi.nlm.nih.gov | |
Description | Data deposited in or computed by PubChem | |
Isomeric SMILES |
CC/C=C\C[C@@H](/C=C/C=C\CCCCCCCC(=O)O)OO | |
Source | PubChem | |
URL | https://pubchem.ncbi.nlm.nih.gov | |
Description | Data deposited in or computed by PubChem | |
Molecular Formula |
C18H30O4 | |
Source | PubChem | |
URL | https://pubchem.ncbi.nlm.nih.gov | |
Description | Data deposited in or computed by PubChem | |
DSSTOX Substance ID |
DTXSID501017285 | |
Record name | 13S-hydroperoxy-9Z,11E,15Z-octadecatrienoic acid | |
Source | EPA DSSTox | |
URL | https://comptox.epa.gov/dashboard/DTXSID501017285 | |
Description | DSSTox provides a high quality public chemistry resource for supporting improved predictive toxicology. | |
Molecular Weight |
310.4 g/mol | |
Source | PubChem | |
URL | https://pubchem.ncbi.nlm.nih.gov | |
Description | Data deposited in or computed by PubChem | |
CAS RN |
67597-26-6 | |
Record name | 13S-hydroperoxy-9Z,11E,15Z-octadecatrienoic acid | |
Source | EPA DSSTox | |
URL | https://comptox.epa.gov/dashboard/DTXSID501017285 | |
Description | DSSTox provides a high quality public chemistry resource for supporting improved predictive toxicology. | |
Record name | 13(S)-Hydroperoxylinolenic acid | |
Source | Human Metabolome Database (HMDB) | |
URL | http://www.hmdb.ca/metabolites/HMDB0301803 | |
Description | The Human Metabolome Database (HMDB) is a freely available electronic database containing detailed information about small molecule metabolites found in the human body. | |
Explanation | HMDB is offered to the public as a freely available resource. Use and re-distribution of the data, in whole or in part, for commercial purposes requires explicit permission of the authors and explicit acknowledgment of the source material (HMDB) and the original publication (see the HMDB citing page). We ask that users who download significant portions of the database cite the HMDB paper in any resulting publications. | |
Retrosynthesis Analysis
AI-Powered Synthesis Planning: Our tool employs the Template_relevance Pistachio, Template_relevance Bkms_metabolic, Template_relevance Pistachio_ringbreaker, Template_relevance Reaxys, Template_relevance Reaxys_biocatalysis model, leveraging a vast database of chemical reactions to predict feasible synthetic routes.
One-Step Synthesis Focus: Specifically designed for one-step synthesis, it provides concise and direct routes for your target compounds, streamlining the synthesis process.
Accurate Predictions: Utilizing the extensive PISTACHIO, BKMS_METABOLIC, PISTACHIO_RINGBREAKER, REAXYS, REAXYS_BIOCATALYSIS database, our tool offers high-accuracy predictions, reflecting the latest in chemical research and data.
Strategy Settings
Precursor scoring | Relevance Heuristic |
---|---|
Min. plausibility | 0.01 |
Model | Template_relevance |
Template Set | Pistachio/Bkms_metabolic/Pistachio_ringbreaker/Reaxys/Reaxys_biocatalysis |
Top-N result to add to graph | 6 |
Feasible Synthetic Routes
Q & A
Q1: How is 13-HPOT synthesized in plants?
A1: 13-HPOT is produced from α-linolenic acid via the action of 13-lipoxygenase (13-LOX) enzymes. This oxygenation reaction is the first committed step in the biosynthesis of jasmonic acid (JA), a critical plant hormone. [, , , , ]
Q2: What are the downstream products of 13-HPOT in plants?
A2: 13-HPOT serves as a substrate for several enzymes, leading to the formation of various bioactive molecules. Allene oxide synthase (AOS) converts 13-HPOT to an unstable allene oxide, which is further cyclized by allene oxide cyclase (AOC) to form 12-oxo-phytodienoic acid (12-OPDA). 12-OPDA is then converted to JA, a key regulator of plant defense and development. [, , , , , , , ]
Q3: Can 13-HPOT be metabolized by other pathways besides the JA pathway?
A3: Yes, 13-HPOT can also be metabolized by hydroperoxide lyase (HPL) to produce volatile aldehydes like hexanal and (Z)-3-hexenal, which contribute to the characteristic aroma of plants upon wounding. These volatiles play a role in attracting pollinators and defending against herbivores. [, , , ]
Q4: Does the presence of specific double bonds in 13-HPOT influence its metabolism?
A4: Yes, the presence of a double bond in the β,γ-position to the hydroperoxide group in 13-HPOT significantly enhances its cyclization rate to form cyclopentenones like 12-oxo-PDA, a crucial precursor to JA. This effect is attributed to anchimeric assistance provided by the double bond. []
Q5: What is known about the regulation of 13-LOX, the enzyme responsible for 13-HPOT synthesis?
A5: 13-LOX activity can be influenced by various factors, including developmental stage, environmental cues, and hormonal signals. For example, wounding in strawberry fruits was shown to increase both LOX and HPL activity, leading to increased production of C6 aldehydes from 13-HPOT. []
Q6: How do environmental factors impact 13-HPOT levels in plants?
A6: Environmental stresses, such as wounding, pathogen attack, and abiotic stresses like drought, can trigger significant changes in 13-HPOT levels. These changes are often associated with the activation of defense responses. [, , , , , ]
Q7: Are there specific inhibitors of enzymes involved in 13-HPOT metabolism?
A7: Yes, diethyldithiocarbamic acid (DIECA) has been shown to inhibit the octadecanoid signaling pathway by reducing 13-HPOT to 13-hydroxylinolenic acid (13-HOTrE), effectively depleting the precursor pool for JA synthesis. [, ]
Q8: What is the role of 13-HPOT in plant defense responses?
A8: 13-HPOT is a key precursor to JA, a central regulator of plant defense against herbivores and pathogens. JA signaling activates the expression of defense-related genes, leading to the production of anti-herbivore and antimicrobial compounds. [, , , , , ]
Q9: Can 13-HPOT be used as a biocontrol agent for plant protection?
A10: Studies have shown that exogenous application of 13-HPOT can enhance plant resistance to certain pathogens. Its potential as a biocontrol agent warrants further exploration. [, ]
Q10: How does 13-HPOT affect plant plasma membrane structure?
A11: Research indicates that 13-HPOT can insert into plant plasma membranes, influencing their fluidity and thickness. This interaction is suggested to contribute to its elicitor activity, triggering early plant defense responses. [, ]
Q11: What is the molecular formula and weight of 13-HPOT?
A11: The molecular formula of 13-HPOT is C18H30O4, and its molecular weight is 306.44 g/mol.
Q12: What are the major spectroscopic techniques used to characterize 13-HPOT?
A13: Commonly used spectroscopic techniques include ultraviolet-visible (UV-Vis) spectroscopy, mass spectrometry (MS), and nuclear magnetic resonance (NMR) spectroscopy. These techniques provide valuable information about the structure and purity of 13-HPOT. [, ]
Q13: What analytical methods are employed to quantify 13-HPOT?
A14: High-performance liquid chromatography (HPLC) is widely used to separate and quantify 13-HPOT from complex mixtures. Different detection methods, such as UV-Vis detectors and mass spectrometers, can be coupled with HPLC to enhance sensitivity and selectivity. [, , ]
Q14: What are some promising areas for future research on 13-HPOT?
A14: Further investigation is needed to fully understand:
試験管内研究製品の免責事項と情報
BenchChemで提示されるすべての記事および製品情報は、情報提供を目的としています。BenchChemで購入可能な製品は、生体外研究のために特別に設計されています。生体外研究は、ラテン語の "in glass" に由来し、生物体の外で行われる実験を指します。これらの製品は医薬品または薬として分類されておらず、FDAから任何の医療状態、病気、または疾患の予防、治療、または治癒のために承認されていません。これらの製品を人間または動物に体内に導入する形態は、法律により厳格に禁止されています。これらのガイドラインに従うことは、研究と実験において法的および倫理的な基準の遵守を確実にするために重要です。