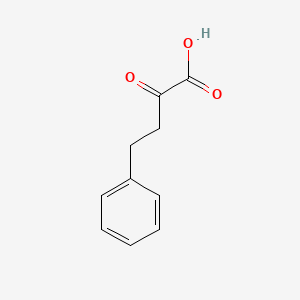
2-Oxo-4-phenylbutyric acid
概要
説明
2-Oxo-4-phenylbutyric acid (OPBA) is an organic compound that is widely used in scientific research, particularly in the field of biochemistry. OPBA is a carboxylic acid that is used as a precursor for the synthesis of other organic compounds and can be used in a variety of laboratory experiments.
科学的研究の応用
Here is a comprehensive analysis of the scientific research applications of 2-oxo-4-phenylbutanoic acid:
Precursor for ACE Inhibitors
This compound serves as an important precursor for the production of a series of angiotensin-converting enzymes (ACE) inhibitors, which are used in drugs like benazepril, cilazapril, quinapril, and ramipril. These drugs contain the (S)-homophenylalanine moiety as a pharmacophore .
Polymorphism Studies
Research has been conducted on different polymorphs of 4-oxo-4-phenylbutanoic acid due to their non-equivalent physical properties and bioactivity. Understanding polymorphism is crucial for pharmaceutical applications due to variations in properties of solids with the same chemical substance but different crystal packing .
Synthesis of Chiral Building Blocks
The compound is used in the synthesis of pharmaceutical chiral building blocks like l-homophenylalanine (l-HPA) through direct reductive amination catalyzed by phenylalanine dehydrogenase (PheDH). This process is attractive because it only uses ammonia and water is the only byproduct .
作用機序
Target of Action
The primary target of 2-oxo-4-phenylbutanoic acid (2-OPBA) is phenylalanine dehydrogenase (PheDH) . PheDH is an enzyme that plays a crucial role in the synthesis of the pharmaceutical chiral building block L-homophenylalanine (L-HPA) . This compound is used in the production of several blockbuster pharmaceuticals, such as angiotensin-converting enzyme inhibitors .
Mode of Action
The interaction of 2-OPBA with its target, PheDH, involves a process called direct reductive amination . In this process, 2-OPBA is catalyzed by PheDH, resulting in the synthesis of L-HPA . The sole expense of this process is ammonia, and water is the only byproduct .
Biochemical Pathways
The biochemical pathway affected by 2-OPBA involves the conversion of 2-OPBA to L-HPA, catalyzed by PheDH . This process is highly attractive in the synthesis of L-HPA, which serves as a versatile chiral building block for the synthesis of several pharmaceuticals .
Pharmacokinetics
It’s worth noting that the biocatalytic process involving 2-opba and phedh has been reported to have high catalytic efficiency .
Result of Action
The result of the action of 2-OPBA is the production of L-HPA . L-HPA is an important precursor for the production of angiotensin-converting enzyme inhibitors, which are significant drugs for preventing the formation of angiotensin II and lowering blood pressure .
Action Environment
The action of 2-OPBA is influenced by environmental factors such as substrate loading and the presence of indispensable cofactors . For instance, the viability of the biocatalytic process involving 2-OPBA and PheDH is markedly affected by insufficient substrate loading and high costs of the indispensable cofactors . A highly efficient and economic biocatalytic process for l-hpa has been established by coupling genetically modified phedh and formate dehydrogenase .
特性
IUPAC Name |
2-oxo-4-phenylbutanoic acid | |
---|---|---|
Source | PubChem | |
URL | https://pubchem.ncbi.nlm.nih.gov | |
Description | Data deposited in or computed by PubChem | |
InChI |
InChI=1S/C10H10O3/c11-9(10(12)13)7-6-8-4-2-1-3-5-8/h1-5H,6-7H2,(H,12,13) | |
Source | PubChem | |
URL | https://pubchem.ncbi.nlm.nih.gov | |
Description | Data deposited in or computed by PubChem | |
InChI Key |
PPKAIMDMNWBOKN-UHFFFAOYSA-N | |
Source | PubChem | |
URL | https://pubchem.ncbi.nlm.nih.gov | |
Description | Data deposited in or computed by PubChem | |
Canonical SMILES |
C1=CC=C(C=C1)CCC(=O)C(=O)O | |
Source | PubChem | |
URL | https://pubchem.ncbi.nlm.nih.gov | |
Description | Data deposited in or computed by PubChem | |
Molecular Formula |
C10H10O3 | |
Source | PubChem | |
URL | https://pubchem.ncbi.nlm.nih.gov | |
Description | Data deposited in or computed by PubChem | |
DSSTOX Substance ID |
DTXSID10221224 | |
Record name | 2-Oxo-4-phenylbutyric acid | |
Source | EPA DSSTox | |
URL | https://comptox.epa.gov/dashboard/DTXSID10221224 | |
Description | DSSTox provides a high quality public chemistry resource for supporting improved predictive toxicology. | |
Molecular Weight |
178.18 g/mol | |
Source | PubChem | |
URL | https://pubchem.ncbi.nlm.nih.gov | |
Description | Data deposited in or computed by PubChem | |
Product Name |
2-Oxo-4-phenylbutyric acid | |
CAS RN |
710-11-2 | |
Record name | α-Oxobenzenebutanoic acid | |
Source | CAS Common Chemistry | |
URL | https://commonchemistry.cas.org/detail?cas_rn=710-11-2 | |
Description | CAS Common Chemistry is an open community resource for accessing chemical information. Nearly 500,000 chemical substances from CAS REGISTRY cover areas of community interest, including common and frequently regulated chemicals, and those relevant to high school and undergraduate chemistry classes. This chemical information, curated by our expert scientists, is provided in alignment with our mission as a division of the American Chemical Society. | |
Explanation | The data from CAS Common Chemistry is provided under a CC-BY-NC 4.0 license, unless otherwise stated. | |
Record name | 2-Oxo-4-phenylbutyric acid | |
Source | ChemIDplus | |
URL | https://pubchem.ncbi.nlm.nih.gov/substance/?source=chemidplus&sourceid=0000710112 | |
Description | ChemIDplus is a free, web search system that provides access to the structure and nomenclature authority files used for the identification of chemical substances cited in National Library of Medicine (NLM) databases, including the TOXNET system. | |
Record name | 2-Oxo-4-phenylbutyric acid | |
Source | EPA DSSTox | |
URL | https://comptox.epa.gov/dashboard/DTXSID10221224 | |
Description | DSSTox provides a high quality public chemistry resource for supporting improved predictive toxicology. | |
Record name | 2-oxo-4-phenylbutyric acid | |
Source | European Chemicals Agency (ECHA) | |
URL | https://echa.europa.eu/substance-information/-/substanceinfo/100.010.834 | |
Description | The European Chemicals Agency (ECHA) is an agency of the European Union which is the driving force among regulatory authorities in implementing the EU's groundbreaking chemicals legislation for the benefit of human health and the environment as well as for innovation and competitiveness. | |
Explanation | Use of the information, documents and data from the ECHA website is subject to the terms and conditions of this Legal Notice, and subject to other binding limitations provided for under applicable law, the information, documents and data made available on the ECHA website may be reproduced, distributed and/or used, totally or in part, for non-commercial purposes provided that ECHA is acknowledged as the source: "Source: European Chemicals Agency, http://echa.europa.eu/". Such acknowledgement must be included in each copy of the material. ECHA permits and encourages organisations and individuals to create links to the ECHA website under the following cumulative conditions: Links can only be made to webpages that provide a link to the Legal Notice page. | |
Synthesis routes and methods I
Procedure details
Synthesis routes and methods II
Procedure details
Synthesis routes and methods III
Procedure details
Retrosynthesis Analysis
AI-Powered Synthesis Planning: Our tool employs the Template_relevance Pistachio, Template_relevance Bkms_metabolic, Template_relevance Pistachio_ringbreaker, Template_relevance Reaxys, Template_relevance Reaxys_biocatalysis model, leveraging a vast database of chemical reactions to predict feasible synthetic routes.
One-Step Synthesis Focus: Specifically designed for one-step synthesis, it provides concise and direct routes for your target compounds, streamlining the synthesis process.
Accurate Predictions: Utilizing the extensive PISTACHIO, BKMS_METABOLIC, PISTACHIO_RINGBREAKER, REAXYS, REAXYS_BIOCATALYSIS database, our tool offers high-accuracy predictions, reflecting the latest in chemical research and data.
Strategy Settings
Precursor scoring | Relevance Heuristic |
---|---|
Min. plausibility | 0.01 |
Model | Template_relevance |
Template Set | Pistachio/Bkms_metabolic/Pistachio_ringbreaker/Reaxys/Reaxys_biocatalysis |
Top-N result to add to graph | 6 |
Feasible Synthetic Routes
Q & A
Q1: What is the molecular formula, weight, and relevant spectroscopic data for 2-Oxo-4-phenylbutyric acid?
A1: 2-Oxo-4-phenylbutyric acid has the molecular formula C10H10O3 and a molecular weight of 178.18 g/mol. While specific spectroscopic data wasn't detailed in the provided research, typical characterization would involve techniques like NMR (Nuclear Magnetic Resonance), IR (Infrared Spectroscopy), and Mass Spectrometry.
Q2: How is 2-Oxo-4-phenylbutyric acid used in the production of pharmaceuticals?
A2: OPBA serves as a prochiral intermediate in the synthesis of ACEI medications []. These medications are widely used for treating hypertension and congestive heart failure.
Q3: Can you elaborate on the enzymatic synthesis of L-Homophenylalanine (L-HPA) using 2-Oxo-4-phenylbutyric acid?
A3: L-HPA, a precursor for ACEIs, can be produced through the reductive amination of OPBA. This reaction is catalyzed by enzymes like L-phenylalanine dehydrogenase [] or meso-diaminopimelate dehydrogenase (DAPDH) [] and often employs a coupled enzyme system for cofactor regeneration [, , ].
Q4: Are there any alternative enzymatic pathways for L-HPA synthesis using OPBA?
A4: Yes, researchers have explored alternative pathways using enzymes like aspartate aminotransferase (AAT) [, , , ]. Engineered E. coli strains expressing modified AAT demonstrated efficient conversion of OPBA to L-HPA using L-lysine as an amino donor [, ].
Q5: What advantages do biocatalytic processes offer in the synthesis of L-HPA from OPBA?
A5: Biocatalytic processes, especially those employing engineered enzymes, offer high enantioselectivity, yielding L-HPA with high optical purity, a critical factor for pharmaceutical applications [, , , , , ].
Q6: How do structural modifications of enzymes involved in OPBA biotransformation affect their activity and selectivity?
A6: Research on enzymes like phenylalanine dehydrogenase (PheDH) highlights the importance of steric hindrance engineering []. Introducing specific mutations within the active site can significantly impact substrate binding and catalytic efficiency. For instance, the triple-site mutant V309G/L306V/V144G in Bacillus badius PheDH showed a remarkable 12.9-fold increase in catalytic efficiency (kcat/Km) compared to the wild-type enzyme [].
Q7: Are there any studies on engineering meso-diaminopimelate dehydrogenase (DAPDH) for improved activity towards OPBA?
A7: Yes, structure-guided mutagenesis of DAPDH from Symbiobacterium thermophilum led to the development of a double-site mutant, W121L/H227I. This mutant displayed significantly enhanced activity towards various 2-keto acids, including bulky substrates like OPBA []. This modification highlights the potential of protein engineering to expand the substrate scope and improve the catalytic efficiency of enzymes for the synthesis of valuable chiral compounds.
Q8: What strategies are employed to enhance the stability and reusability of enzymes used in OPBA biotransformation?
A8: Immobilization of enzymes, such as D-lactate dehydrogenase (D-nLDH) and formate dehydrogenase (FDH), onto solid supports like cotton cloth has been investigated to improve stability and facilitate continuous processes []. This approach allows for enzyme reuse and simplifies downstream processing.
Q9: How is 2-Oxo-4-phenylbutyric acid typically synthesized, and how is the reaction optimized?
A9: A common method involves the condensation of ethyl 3-phenylpropionate with diethyl oxalate, followed by hydrolysis []. Optimization often focuses on parameters like reactant ratios, reaction times, and temperature, as highlighted in studies aiming for high OPBA yields [, ].
Q10: Are there alternative routes or starting materials for the synthesis of compounds typically derived from 2-Oxo-4-phenylbutyric acid?
A10: While the provided research focuses primarily on OPBA as a key intermediate, exploring alternative synthetic routes for ACE inhibitors is an active area of research. Direct asymmetric hydrogenation of related compounds, like 2-oxo-4-arylbut-3-enoic acids, has shown promise in producing 2-hydroxy-4-arylbutanoic acids, which are also intermediates for ACE inhibitors [].
試験管内研究製品の免責事項と情報
BenchChemで提示されるすべての記事および製品情報は、情報提供を目的としています。BenchChemで購入可能な製品は、生体外研究のために特別に設計されています。生体外研究は、ラテン語の "in glass" に由来し、生物体の外で行われる実験を指します。これらの製品は医薬品または薬として分類されておらず、FDAから任何の医療状態、病気、または疾患の予防、治療、または治癒のために承認されていません。これらの製品を人間または動物に体内に導入する形態は、法律により厳格に禁止されています。これらのガイドラインに従うことは、研究と実験において法的および倫理的な基準の遵守を確実にするために重要です。