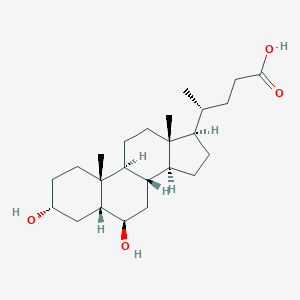
Murideoxycholic acid
概要
説明
Murideoxycholic acid (MDCA), chemically known as 3α,6β-dihydroxy-5β-cholanoic acid (C24H40O4), is a secondary bile acid primarily studied in rodents but also detected in human metabolism . It is characterized by hydroxyl groups at the 3α and 6β positions, distinguishing it from other bile acids like ursodeoxycholic acid (UDCA) and hyodeoxycholic acid (HDCA) . MDCA is synthesized via hepatic modification of primary bile acids such as α- and β-muricholic acids (MCAs) in rodents, and its formation involves microbial dehydroxylation in the gut .
MDCA has been implicated in cholesterol gallstone remodeling, gut microbiota interactions, and metabolic regulation. Notably, it forms insoluble calcium salts (e.g., murideoxycholyl taurine) in gallstones, a unique property that differentiates it from other hydrophilic bile acids . Its role in humans remains less defined but is linked to lipid metabolism and microbiota dysbiosis in conditions like metabolic dysfunction-associated fatty liver disease (MAFLD) .
準備方法
ムロコール酸は、ケノデオキシコール酸から複数段階を経て合成できる。 例えば、α-ムロコール酸は、ケノデオキシコール酸から9段階のプロセスを経て合成でき、全体の収率は26%である 。 工業的な生産方法では、シトクロムP450酵素(特にCyp2c70)を用いて、げっ歯類においてムリコラートを形成する6-ヒドロキシル化反応を触媒する .
化学反応の分析
Biotransformation of Murideoxycholic Acid
MDCA undergoes several metabolic transformations, primarily through hydroxylation and oxidation reactions.
-
Further Biotransformation : MDCA can undergo further biotransformation in the gut, potentially leading to more complex bile acid derivatives.
-
Epimerization : Hydroxysteroid dehydrogenases (HSDHs) catalyze the epimerization of hydroxyl groups. CDCA and CA are epimerized into UDCA and ursocholic acid (UCA) and, subsequently, into isoUDCA and isoUCA, respectively .
Role of Gut Microbiota
Intestinal bacteria play a significant role in the metabolism of bile acids like MDCA .
-
Dehydroxylation : Primary bile acids are dehydroxylated by gut bacteria.
-
Bile Salt Hydrolases (BSHs) : BSHs from the choloylglycine hydrolase family facilitate the deconjugation of bile acids, forming unconjugated bile acids and free glycine or taurine .
-
7α-dehydroxylation : The removal of glycine/taurine by BSHs is a prerequisite for 7α-dehydroxylation by intestinal bacteria.
Oxidation of Bile Acids
Electrochemical oxidation can be used to generate oxidation products of primary bile acids such as cholic acid and chenodeoxycholic acid .
Metabolism and Excretion
This compound (MDCA) is metabolized and excreted in humans .
-
A study was conducted on patients, some with cystic duct drains and others with intact enterohepatic circulation, who ingested 3H-MDCA. The time-course of radioactivity in plasma was followed, and biliary, urinary, and fecal excretions of radioactivity were measured. The excreted MDCA metabolites were then identified .
-
The excretion of radioactivity reached 97.8% in cholecystectomized patients via bile, urine, and feces, but dropped to 75% in patients with lithiasis (urine + feces) .
-
The biological half-life of MDCA in lithiasic patients averaged 3.4 days .
Biological Activities of this compound
MDCA exhibits several biological activities:
-
Fat Digestion and Absorption : MDCA plays a role in fat digestion and absorption by forming micelles, which solubilize dietary fats and facilitate their transport across the intestinal wall.
-
Influence on Gut Microbiota : MDCA may influence gut microbiota composition.
Comparison with Other Bile Acids
This compound shares structural similarities with other bile acids:
Compound Name | Structural Characteristics | Unique Features |
---|---|---|
Chenodeoxycholic Acid | 3α-hydroxy steroid with two additional hydroxyls | Primary bile acid with significant metabolic roles |
Deoxycholic Acid | 3α-hydroxy steroid with one less hydroxyl | Known for its role in cholesterol metabolism |
Ursodeoxycholic Acid | 3α-hydroxy steroid with an additional hydroxyl at position 7 | Used therapeutically to dissolve gallstones |
Lithocholic Acid | 3α-hydroxy steroid lacking hydroxyls at positions 6 and 7 | More toxic than other bile acids |
科学的研究の応用
2.1. Neuroprotective Effects
MDCA has shown promise in neuroprotection, particularly in models of neurodegenerative diseases. Studies indicate that MDCA may alleviate endoplasmic reticulum (ER) stress and apoptosis in neuronal cells, which are critical factors in conditions like Huntington's disease and Alzheimer's disease.
- Case Study : In a transgenic mouse model of Huntington's disease, MDCA treatment resulted in reduced striatal atrophy and improved locomotor function, suggesting its potential as a therapeutic agent for neurodegenerative disorders .
2.2. Metabolic Disorders
Research has indicated that MDCA can influence metabolic pathways, particularly related to glucose metabolism and insulin sensitivity.
- Case Study : In diabetic mouse models, MDCA administration significantly reduced blood glucose levels and albuminuria while improving renal histopathology. These effects were associated with decreased expression of ER stress markers .
2.3. Cardiovascular Health
MDCA's role in cardiovascular health has been explored, particularly concerning obesity-induced cardiac dysfunction.
- Case Study : In obese mice, chronic treatment with MDCA improved myocardial contractile function and reduced systolic blood pressure, highlighting its potential as a therapeutic target for obesity-related cardiac issues .
Comparative Data Table
The following table summarizes key findings from studies investigating the applications of MDCA:
作用機序
ムロコール酸の作用機序は、FXRなどの胆汁酸受容体との相互作用に関与している。 例えば、タウロムリコール酸は、胆汁酸合成、代謝、輸送に関与する遺伝子の発現を調節するFXRの拮抗剤として作用する 。 この相互作用は、胆汁酸蓄積の有害な影響を最小限に抑えるのに役立つ 。 Cyp2c70酵素は、ケノデオキシコール酸やウルソデオキシコール酸からムリコール酸を生成する6-ヒドロキシル化反応を担っている .
類似の化合物との比較
ムロコール酸は、コール酸、ケノデオキシコール酸、ウルソデオキシコール酸などの他の胆汁酸に似ている。 6位にβ配置の水酸基を持つ点が特徴 。この構造的な違いにより、ムロコール酸は、他の胆汁酸とは異なる性質と機能を持つ。 類似の化合物には、α-ムリコール酸、β-ムリコール酸、γ-ムリコール酸(ヒオコール酸)、ω-ムリコール酸などがある .
類似化合物との比較
Comparison with Structurally and Functionally Similar Bile Acids
Structural Comparison
Key structural differences between MDCA and related bile acids are summarized below:
Key Insights :
- MDCA’s 6β-hydroxyl group contributes to its unique insolubility and propensity to form calcium salts, unlike UDCA or β-muricholic acid .
- Compared to HDCA (6α-hydroxyl), MDCA’s 6β configuration reduces its detergent properties, impacting micelle formation and cholesterol solubilization .
Functional and Metabolic Differences
Gallstone Remodeling
- UDCA : Dissolves cholesterol gallstones by reducing cholesterol secretion and promoting micellar solubility .
Metabolic Pathways
- MDCA : A secondary bile acid in rodents, derived from α/β-MCA via gut microbiota . In humans, MDCA levels correlate negatively with MAFLD severity, suggesting a regulatory role in lipid homeostasis .
- Deoxycholic Acid (DCA) : Formed from CA via 7α-dehydroxylation; promotes colorectal cancer via DNA damage, contrasting with MDCA’s neutral oncogenic profile .
Microbiota Interactions
- MDCA and HDCA are strongly associated with Clostridium species, which mediate their synthesis .
- MDCA levels decrease in type 2 diabetes mellitus (T2DM) models but are restored by herbal interventions like GGQLT, indicating microbiota-dependent regulation .
Species-Specific Variations
Aspect | Rodents | Humans |
---|---|---|
Primary BAs | CA, α/β-MCA | CA, CDCA |
Secondary BAs | MDCA, HDCA, ω-MCA | DCA, LCA, UDCA |
MDCA Synthesis | Hepatic and microbial modification of MCA | Trace amounts detected in fecal samples |
Implications : MDCA’s role in cholesterol gallstone formation is prominent in rodents but less relevant in humans, where UDCA and TUDCA are therapeutic mainstays .
Research Findings and Clinical Relevance
Key Studies on MDCA
Gallstone Remodeling (1995) : MDCA replaced cholesterol gallstones with insoluble salts in prairie dogs, highlighting its lack of solubilizing efficacy compared to UDCA .
Metabolic Excretion (1998) : In humans, 60% of orally administered MDCA was excreted in feces within 72 hours, with minimal urinary excretion, underscoring its poor systemic absorption .
生物活性
Murideoxycholic acid (MDCA) is a secondary bile acid predominantly found in mice, derived from α- and β-muricholic acids. It plays a significant role in various biological processes, particularly in lipid metabolism, gut microbiota modulation, and potential therapeutic applications. This article delves into the biological activity of MDCA, highlighting its metabolic pathways, physiological effects, and relevant case studies.
Metabolism and Biosynthesis
MDCA is synthesized in the intestine from primary bile acids through microbial action. Research indicates that MDCA is a pivotal metabolite involved in the enterohepatic circulation of bile acids, influencing both lipid absorption and metabolism. The metabolic pathways of MDCA include:
- Conversion from Primary Bile Acids : MDCA is formed from α- and β-muricholic acids through enzymatic processes facilitated by gut microbiota.
- Oxidation Reactions : Studies have shown that MDCA participates in various oxidation reactions, which are crucial for its biological activity. For instance, the oxidation of MDCA can lead to the formation of other bioactive metabolites that further influence metabolic pathways in mice .
Physiological Effects
MDCA exhibits several physiological effects that contribute to its biological activity:
- Lipid Metabolism : MDCA has been shown to enhance lipid absorption and metabolism by activating specific nuclear receptors involved in lipid homeostasis, such as FXR (Farnesoid X receptor) and LXR (Liver X receptor) .
- Gut Microbiota Modulation : As a bile acid, MDCA influences the composition of gut microbiota, promoting beneficial bacteria while inhibiting pathogenic strains. This modulation can have significant implications for metabolic health and disease prevention .
- Neuroprotective Properties : Emerging studies suggest that MDCA may possess neuroprotective effects similar to other bile acids. It has been implicated in reducing oxidative stress and inflammation in neuronal tissues .
Case Study 1: Impact on Lipid Metabolism
A study investigating the effects of MDCA on lipid metabolism in mice demonstrated that administration of MDCA significantly increased the levels of beneficial lipids while decreasing harmful lipid species. The results indicated enhanced expression of genes involved in fatty acid oxidation and reduced hepatic steatosis .
Case Study 2: Gut Microbiota Interaction
In another study focusing on the interaction between MDCA and gut microbiota, researchers observed that supplementation with MDCA altered the microbial composition favorably. Mice treated with MDCA showed an increase in beneficial bacteria such as Lactobacillus spp., which are known for their positive effects on gut health and immune function .
Table 1: Summary of Biological Activities of MDCA
Biological Activity | Description |
---|---|
Lipid Metabolism | Enhances lipid absorption; activates FXR and LXR receptors |
Gut Microbiota Modulation | Promotes beneficial bacteria; inhibits pathogenic strains |
Neuroprotective Effects | Reduces oxidative stress; potential protection against neurodegeneration |
Table 2: Key Findings from Case Studies
Study Focus | Key Findings |
---|---|
Lipid Metabolism | Increased beneficial lipids; reduced hepatic steatosis |
Gut Microbiota Interaction | Altered microbial composition; increased Lactobacillus spp. |
Q & A
Basic Research Questions
Q. What are the primary methods for synthesizing MDCA in laboratory settings?
MDCA can be synthesized via enzymatic and microbial pathways. The bacterial P450 monooxygenase CYP107D1 (OleP) selectively hydroxylates lithocholic acid (LCA) to MDCA with a 19.5% yield in whole-cell biotransformations . Alternatively, microbial 7-dehydroxylation of α-muricholic acid (α-MCA) in rodent models produces MDCA, mediated by gut microbiota such as Clostridium and Eubacterium species .
Q. Which analytical techniques are recommended for identifying and quantifying MDCA in biological samples?
- Nuclear Magnetic Resonance (NMR) : Used to confirm structural identity, particularly for distinguishing MDCA from isomers .
- LC-MS/MS with MRM technology : Provides high sensitivity for quantification in complex matrices like serum or fecal samples .
- Chromatographic separation : Optimize reverse-phase columns to resolve MDCA from co-eluting bile acids .
Q. What experimental models are commonly used to study MDCA's metabolic roles?
- Rodent models : MDCA is a secondary bile acid in mice, derived from α-MCA via microbial metabolism .
- In vitro systems : Hepatic microsomes (e.g., from rats) are used to study CYP enzyme-mediated MDCA synthesis .
Advanced Research Questions
Q. How can researchers reconcile discrepancies in enzymatic synthesis yields of MDCA?
Variability in yields (e.g., 19.5% for CYP107D1 vs. lower yields in microsomal systems) may arise from differences in enzyme activity, substrate concentration, or reaction conditions. Optimization strategies include:
- Adjusting NADPH cofactor levels in microsomal assays .
- Using engineered bacterial strains to enhance CYP107D1 expression .
- Monitoring reaction kinetics to identify rate-limiting steps .
Q. What mechanisms explain MDCA's role in modulating metabolic pathways in vivo?
MDCA influences bile acid homeostasis by:
- Competing with other bile acids for reabsorption via the ASBT transporter in the ileum .
- Modulating farnesoid X receptor (FXR) signaling, though its affinity is lower than primary bile acids .
- Altering gut microbiota composition through bacteriostatic effects .
Q. How should researchers address contradictions in safety guidelines for handling MDCA?
While some safety data sheets (SDS) state "no special measures required" , others classify MDCA as a skin/eye irritant (Category 2/2A) . To mitigate risks:
- Use nitrile gloves and safety goggles during handling .
- Store MDCA at -20°C under inert atmosphere to prevent degradation .
- Follow institutional protocols for waste disposal to avoid environmental contamination .
Q. Methodological and Data Analysis Considerations
Q. What steps ensure the purity and identity of MDCA in experimental samples?
- Purity validation : Use HPLC with UV detection (λ = 210 nm) and compare retention times to certified standards .
- Structural confirmation : Combine high-resolution mass spectrometry (HRMS) with 1H/13C NMR for unambiguous identification .
Q. How can variability in CYP enzyme activity be controlled during MDCA synthesis?
- Pre-treat animal models with CYP inducers (e.g., phenobarbital for CYP2C/3A enzymes) to enhance reproducibility .
- Include control reactions without NADPH to confirm enzyme-dependent synthesis .
Q. What strategies improve LC-MS/MS detection limits for MDCA in low-abundance samples?
- Derivatize MDCA with charged moieties (e.g., Girard’s reagent) to enhance ionization efficiency .
- Use isotope-labeled internal standards (e.g., d4-MDCA) to correct for matrix effects .
Q. Contradictions and Emerging Research Directions
特性
IUPAC Name |
(4R)-4-[(3R,5R,6R,8S,9S,10R,13R,14S,17R)-3,6-dihydroxy-10,13-dimethyl-2,3,4,5,6,7,8,9,11,12,14,15,16,17-tetradecahydro-1H-cyclopenta[a]phenanthren-17-yl]pentanoic acid | |
---|---|---|
Source | PubChem | |
URL | https://pubchem.ncbi.nlm.nih.gov | |
Description | Data deposited in or computed by PubChem | |
InChI |
InChI=1S/C24H40O4/c1-14(4-7-22(27)28)17-5-6-18-16-13-21(26)20-12-15(25)8-10-24(20,3)19(16)9-11-23(17,18)2/h14-21,25-26H,4-13H2,1-3H3,(H,27,28)/t14-,15-,16+,17-,18+,19+,20+,21-,23-,24-/m1/s1 | |
Source | PubChem | |
URL | https://pubchem.ncbi.nlm.nih.gov | |
Description | Data deposited in or computed by PubChem | |
InChI Key |
DGABKXLVXPYZII-PLYQRAMGSA-N | |
Source | PubChem | |
URL | https://pubchem.ncbi.nlm.nih.gov | |
Description | Data deposited in or computed by PubChem | |
Canonical SMILES |
CC(CCC(=O)O)C1CCC2C1(CCC3C2CC(C4C3(CCC(C4)O)C)O)C | |
Source | PubChem | |
URL | https://pubchem.ncbi.nlm.nih.gov | |
Description | Data deposited in or computed by PubChem | |
Isomeric SMILES |
C[C@H](CCC(=O)O)[C@H]1CC[C@@H]2[C@@]1(CC[C@H]3[C@H]2C[C@H]([C@H]4[C@@]3(CC[C@H](C4)O)C)O)C | |
Source | PubChem | |
URL | https://pubchem.ncbi.nlm.nih.gov | |
Description | Data deposited in or computed by PubChem | |
Molecular Formula |
C24H40O4 | |
Source | PubChem | |
URL | https://pubchem.ncbi.nlm.nih.gov | |
Description | Data deposited in or computed by PubChem | |
DSSTOX Substance ID |
DTXSID40861652 | |
Record name | Murideoxycholic acid | |
Source | EPA DSSTox | |
URL | https://comptox.epa.gov/dashboard/DTXSID40861652 | |
Description | DSSTox provides a high quality public chemistry resource for supporting improved predictive toxicology. | |
Molecular Weight |
392.6 g/mol | |
Source | PubChem | |
URL | https://pubchem.ncbi.nlm.nih.gov | |
Description | Data deposited in or computed by PubChem | |
Physical Description |
Solid | |
Record name | Murocholic acid | |
Source | Human Metabolome Database (HMDB) | |
URL | http://www.hmdb.ca/metabolites/HMDB0000811 | |
Description | The Human Metabolome Database (HMDB) is a freely available electronic database containing detailed information about small molecule metabolites found in the human body. | |
Explanation | HMDB is offered to the public as a freely available resource. Use and re-distribution of the data, in whole or in part, for commercial purposes requires explicit permission of the authors and explicit acknowledgment of the source material (HMDB) and the original publication (see the HMDB citing page). We ask that users who download significant portions of the database cite the HMDB paper in any resulting publications. | |
CAS No. |
668-49-5 | |
Record name | Murideoxycholic acid | |
Source | CAS Common Chemistry | |
URL | https://commonchemistry.cas.org/detail?cas_rn=668-49-5 | |
Description | CAS Common Chemistry is an open community resource for accessing chemical information. Nearly 500,000 chemical substances from CAS REGISTRY cover areas of community interest, including common and frequently regulated chemicals, and those relevant to high school and undergraduate chemistry classes. This chemical information, curated by our expert scientists, is provided in alignment with our mission as a division of the American Chemical Society. | |
Explanation | The data from CAS Common Chemistry is provided under a CC-BY-NC 4.0 license, unless otherwise stated. | |
Record name | Murideoxycholic acid | |
Source | ChemIDplus | |
URL | https://pubchem.ncbi.nlm.nih.gov/substance/?source=chemidplus&sourceid=0000668495 | |
Description | ChemIDplus is a free, web search system that provides access to the structure and nomenclature authority files used for the identification of chemical substances cited in National Library of Medicine (NLM) databases, including the TOXNET system. | |
Record name | Murideoxycholic acid | |
Source | EPA DSSTox | |
URL | https://comptox.epa.gov/dashboard/DTXSID40861652 | |
Description | DSSTox provides a high quality public chemistry resource for supporting improved predictive toxicology. | |
Record name | MURIDEOXYCHOLIC ACID | |
Source | FDA Global Substance Registration System (GSRS) | |
URL | https://gsrs.ncats.nih.gov/ginas/app/beta/substances/AMB87E8LOH | |
Description | The FDA Global Substance Registration System (GSRS) enables the efficient and accurate exchange of information on what substances are in regulated products. Instead of relying on names, which vary across regulatory domains, countries, and regions, the GSRS knowledge base makes it possible for substances to be defined by standardized, scientific descriptions. | |
Explanation | Unless otherwise noted, the contents of the FDA website (www.fda.gov), both text and graphics, are not copyrighted. They are in the public domain and may be republished, reprinted and otherwise used freely by anyone without the need to obtain permission from FDA. Credit to the U.S. Food and Drug Administration as the source is appreciated but not required. | |
Record name | Murocholic acid | |
Source | Human Metabolome Database (HMDB) | |
URL | http://www.hmdb.ca/metabolites/HMDB0000811 | |
Description | The Human Metabolome Database (HMDB) is a freely available electronic database containing detailed information about small molecule metabolites found in the human body. | |
Explanation | HMDB is offered to the public as a freely available resource. Use and re-distribution of the data, in whole or in part, for commercial purposes requires explicit permission of the authors and explicit acknowledgment of the source material (HMDB) and the original publication (see the HMDB citing page). We ask that users who download significant portions of the database cite the HMDB paper in any resulting publications. | |
Retrosynthesis Analysis
AI-Powered Synthesis Planning: Our tool employs the Template_relevance Pistachio, Template_relevance Bkms_metabolic, Template_relevance Pistachio_ringbreaker, Template_relevance Reaxys, Template_relevance Reaxys_biocatalysis model, leveraging a vast database of chemical reactions to predict feasible synthetic routes.
One-Step Synthesis Focus: Specifically designed for one-step synthesis, it provides concise and direct routes for your target compounds, streamlining the synthesis process.
Accurate Predictions: Utilizing the extensive PISTACHIO, BKMS_METABOLIC, PISTACHIO_RINGBREAKER, REAXYS, REAXYS_BIOCATALYSIS database, our tool offers high-accuracy predictions, reflecting the latest in chemical research and data.
Strategy Settings
Precursor scoring | Relevance Heuristic |
---|---|
Min. plausibility | 0.01 |
Model | Template_relevance |
Template Set | Pistachio/Bkms_metabolic/Pistachio_ringbreaker/Reaxys/Reaxys_biocatalysis |
Top-N result to add to graph | 6 |
Feasible Synthetic Routes
試験管内研究製品の免責事項と情報
BenchChemで提示されるすべての記事および製品情報は、情報提供を目的としています。BenchChemで購入可能な製品は、生体外研究のために特別に設計されています。生体外研究は、ラテン語の "in glass" に由来し、生物体の外で行われる実験を指します。これらの製品は医薬品または薬として分類されておらず、FDAから任何の医療状態、病気、または疾患の予防、治療、または治癒のために承認されていません。これらの製品を人間または動物に体内に導入する形態は、法律により厳格に禁止されています。これらのガイドラインに従うことは、研究と実験において法的および倫理的な基準の遵守を確実にするために重要です。