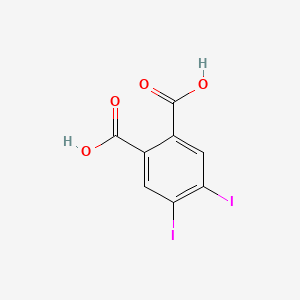
4,5-Diiodo-phthalic acid
説明
4,5-Diiodo-phthalic acid, also known as Dipic, is a chemical compound with the molecular formula C8H4I2O4. It is a white crystalline powder that is soluble in water and organic solvents. Dipic has been widely used in scientific research due to its unique properties and potential applications in various fields.
作用機序
The mechanism of action of 4,5-Diiodo-phthalic acid is not fully understood, but it is believed to be due to its ability to form strong coordination bonds with metal ions. This allows for the formation of various metal complexes, which can exhibit unique properties and functions. The coordination of 4,5-Diiodo-phthalic acid with metal ions can also affect the reactivity and stability of the metal complexes.
Biochemical and Physiological Effects:
There is limited research on the biochemical and physiological effects of 4,5-Diiodo-phthalic acid. However, some studies have suggested that it may have potential applications in the treatment of cancer and other diseases. 4,5-Diiodo-phthalic acid has been shown to inhibit the growth of cancer cells in vitro, and it has been studied as a potential anticancer agent.
実験室実験の利点と制限
The advantages of using 4,5-Diiodo-phthalic acid in lab experiments include its high purity, solubility in water and organic solvents, and ability to form strong coordination bonds with metal ions. However, 4,5-Diiodo-phthalic acid has some limitations, including its high cost, toxicity, and limited availability.
将来の方向性
There are many future directions for the use of 4,5-Diiodo-phthalic acid in scientific research. Some potential areas of research include the synthesis of new metal-organic frameworks and coordination polymers with unique properties and functions, the development of new metal complexes for catalysis and sensing applications, and the study of 4,5-Diiodo-phthalic acid as a potential therapeutic agent for the treatment of cancer and other diseases. Further research is needed to fully understand the potential applications of 4,5-Diiodo-phthalic acid in various fields.
科学的研究の応用
4,5-Diiodo-phthalic acid has been extensively used in scientific research as a building block for the synthesis of various organic compounds. It has been used in the synthesis of metal-organic frameworks (MOFs) and coordination polymers, which have potential applications in gas storage, catalysis, and drug delivery. 4,5-Diiodo-phthalic acid has also been used as a ligand in the synthesis of metal complexes, which have been studied for their magnetic and optical properties.
特性
IUPAC Name |
4,5-diiodophthalic acid | |
---|---|---|
Source | PubChem | |
URL | https://pubchem.ncbi.nlm.nih.gov | |
Description | Data deposited in or computed by PubChem | |
InChI |
InChI=1S/C8H4I2O4/c9-5-1-3(7(11)12)4(8(13)14)2-6(5)10/h1-2H,(H,11,12)(H,13,14) | |
Source | PubChem | |
URL | https://pubchem.ncbi.nlm.nih.gov | |
Description | Data deposited in or computed by PubChem | |
InChI Key |
RWFDZJFUBBFWKQ-UHFFFAOYSA-N | |
Source | PubChem | |
URL | https://pubchem.ncbi.nlm.nih.gov | |
Description | Data deposited in or computed by PubChem | |
Canonical SMILES |
C1=C(C(=CC(=C1I)I)C(=O)O)C(=O)O | |
Source | PubChem | |
URL | https://pubchem.ncbi.nlm.nih.gov | |
Description | Data deposited in or computed by PubChem | |
Molecular Formula |
C8H4I2O4 | |
Source | PubChem | |
URL | https://pubchem.ncbi.nlm.nih.gov | |
Description | Data deposited in or computed by PubChem | |
DSSTOX Substance ID |
DTXSID00449036 | |
Record name | 4,5-DIIODO-PHTHALIC ACID | |
Source | EPA DSSTox | |
URL | https://comptox.epa.gov/dashboard/DTXSID00449036 | |
Description | DSSTox provides a high quality public chemistry resource for supporting improved predictive toxicology. | |
Molecular Weight |
417.92 g/mol | |
Source | PubChem | |
URL | https://pubchem.ncbi.nlm.nih.gov | |
Description | Data deposited in or computed by PubChem | |
Product Name |
4,5-Diiodo-phthalic acid | |
CAS RN |
82679-28-5 | |
Record name | 4,5-DIIODO-PHTHALIC ACID | |
Source | EPA DSSTox | |
URL | https://comptox.epa.gov/dashboard/DTXSID00449036 | |
Description | DSSTox provides a high quality public chemistry resource for supporting improved predictive toxicology. | |
Retrosynthesis Analysis
AI-Powered Synthesis Planning: Our tool employs the Template_relevance Pistachio, Template_relevance Bkms_metabolic, Template_relevance Pistachio_ringbreaker, Template_relevance Reaxys, Template_relevance Reaxys_biocatalysis model, leveraging a vast database of chemical reactions to predict feasible synthetic routes.
One-Step Synthesis Focus: Specifically designed for one-step synthesis, it provides concise and direct routes for your target compounds, streamlining the synthesis process.
Accurate Predictions: Utilizing the extensive PISTACHIO, BKMS_METABOLIC, PISTACHIO_RINGBREAKER, REAXYS, REAXYS_BIOCATALYSIS database, our tool offers high-accuracy predictions, reflecting the latest in chemical research and data.
Strategy Settings
Precursor scoring | Relevance Heuristic |
---|---|
Min. plausibility | 0.01 |
Model | Template_relevance |
Template Set | Pistachio/Bkms_metabolic/Pistachio_ringbreaker/Reaxys/Reaxys_biocatalysis |
Top-N result to add to graph | 6 |
Feasible Synthetic Routes
試験管内研究製品の免責事項と情報
BenchChemで提示されるすべての記事および製品情報は、情報提供を目的としています。BenchChemで購入可能な製品は、生体外研究のために特別に設計されています。生体外研究は、ラテン語の "in glass" に由来し、生物体の外で行われる実験を指します。これらの製品は医薬品または薬として分類されておらず、FDAから任何の医療状態、病気、または疾患の予防、治療、または治癒のために承認されていません。これらの製品を人間または動物に体内に導入する形態は、法律により厳格に禁止されています。これらのガイドラインに従うことは、研究と実験において法的および倫理的な基準の遵守を確実にするために重要です。