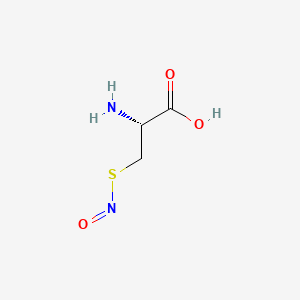
S-Nitrosocysteine
概要
説明
S-Nitrosocysteine is a compound formed by the covalent attachment of a nitrogen monoxide group to the thiol side chain of cysteineThis compound is involved in various physiological processes, including vasodilation, neurotransmission, and immune response regulation .
準備方法
Synthetic Routes and Reaction Conditions: S-Nitrosocysteine can be synthesized through several methods. One common approach involves the reaction of cysteine with nitrous acid, which is generated in situ from sodium nitrite and hydrochloric acid. The reaction typically occurs under acidic conditions and at low temperatures to prevent the decomposition of the product .
Industrial Production Methods: Industrial production of this compound often involves the use of more controlled and scalable processes. One such method includes the use of nitric oxide donors, such as S-nitrosoglutathione, which can transfer the nitrogen monoxide group to cysteine under mild conditions .
化学反応の分析
Types of Reactions: S-Nitrosocysteine undergoes various chemical reactions, including:
Oxidation: this compound can be oxidized to form cysteine sulfenic acid, sulfinic acid, or sulfonic acid.
Reduction: The compound can be reduced back to cysteine by reducing agents such as ascorbate.
Transnitrosation: this compound can transfer its nitrogen monoxide group to other thiols, forming new S-nitrosothiols.
Common Reagents and Conditions:
Oxidation: Hydrogen peroxide or other oxidizing agents under neutral to slightly acidic conditions.
Reduction: Ascorbate or other reducing agents under neutral to slightly basic conditions.
Transnitrosation: S-nitrosoglutathione or other S-nitrosothiols under physiological conditions.
Major Products:
Oxidation: Cysteine sulfenic acid, sulfinic acid, sulfonic acid.
Reduction: Cysteine.
Transnitrosation: New S-nitrosothiols.
科学的研究の応用
S-Nitrosocysteine has a wide range of applications in scientific research:
作用機序
S-Nitrosocysteine exerts its effects primarily through the process of S-nitrosylation, where the nitrogen monoxide group is covalently attached to the thiol side chain of cysteine residues in proteins. This modification can alter the protein’s activity, localization, and interactions. The molecular targets of this compound include various enzymes, receptors, and ion channels. The pathways involved in its action are diverse and include the regulation of blood flow, neurotransmission, and immune responses .
類似化合物との比較
S-Nitrosoglutathione: Another S-nitrosothiol that acts as a nitric oxide donor and has similar biological activities.
S-Nitrosothiols: A broader class of compounds that include S-Nitrosocysteine and S-Nitrosoglutathione, all of which participate in nitric oxide signaling.
Uniqueness of this compound: this compound is unique due to its specific role in protein S-nitrosylation, which directly impacts protein function and cellular signaling. Unlike other S-nitrosothiols, this compound is directly involved in the modification of cysteine residues in proteins, making it a critical player in nitric oxide-mediated signaling pathways .
特性
IUPAC Name |
(2R)-2-amino-3-nitrososulfanylpropanoic acid | |
---|---|---|
Source | PubChem | |
URL | https://pubchem.ncbi.nlm.nih.gov | |
Description | Data deposited in or computed by PubChem | |
InChI |
InChI=1S/C3H6N2O3S/c4-2(3(6)7)1-9-5-8/h2H,1,4H2,(H,6,7)/t2-/m0/s1 | |
Source | PubChem | |
URL | https://pubchem.ncbi.nlm.nih.gov | |
Description | Data deposited in or computed by PubChem | |
InChI Key |
XOWVFANEOZMPKG-REOHCLBHSA-N | |
Source | PubChem | |
URL | https://pubchem.ncbi.nlm.nih.gov | |
Description | Data deposited in or computed by PubChem | |
Canonical SMILES |
C(C(C(=O)O)N)SN=O | |
Source | PubChem | |
URL | https://pubchem.ncbi.nlm.nih.gov | |
Description | Data deposited in or computed by PubChem | |
Isomeric SMILES |
C([C@@H](C(=O)O)N)SN=O | |
Source | PubChem | |
URL | https://pubchem.ncbi.nlm.nih.gov | |
Description | Data deposited in or computed by PubChem | |
Molecular Formula |
C3H6N2O3S | |
Source | PubChem | |
URL | https://pubchem.ncbi.nlm.nih.gov | |
Description | Data deposited in or computed by PubChem | |
Molecular Weight |
150.16 g/mol | |
Source | PubChem | |
URL | https://pubchem.ncbi.nlm.nih.gov | |
Description | Data deposited in or computed by PubChem | |
CAS No. |
51209-75-7 | |
Record name | S-Nitrosocysteine | |
Source | CAS Common Chemistry | |
URL | https://commonchemistry.cas.org/detail?cas_rn=51209-75-7 | |
Description | CAS Common Chemistry is an open community resource for accessing chemical information. Nearly 500,000 chemical substances from CAS REGISTRY cover areas of community interest, including common and frequently regulated chemicals, and those relevant to high school and undergraduate chemistry classes. This chemical information, curated by our expert scientists, is provided in alignment with our mission as a division of the American Chemical Society. | |
Explanation | The data from CAS Common Chemistry is provided under a CC-BY-NC 4.0 license, unless otherwise stated. | |
Record name | S-Nitrosocysteine | |
Source | ChemIDplus | |
URL | https://pubchem.ncbi.nlm.nih.gov/substance/?source=chemidplus&sourceid=0051209757 | |
Description | ChemIDplus is a free, web search system that provides access to the structure and nomenclature authority files used for the identification of chemical substances cited in National Library of Medicine (NLM) databases, including the TOXNET system. | |
Record name | S-NITROSOCYSTEINE | |
Source | FDA Global Substance Registration System (GSRS) | |
URL | https://gsrs.ncats.nih.gov/ginas/app/beta/substances/926P2322P4 | |
Description | The FDA Global Substance Registration System (GSRS) enables the efficient and accurate exchange of information on what substances are in regulated products. Instead of relying on names, which vary across regulatory domains, countries, and regions, the GSRS knowledge base makes it possible for substances to be defined by standardized, scientific descriptions. | |
Explanation | Unless otherwise noted, the contents of the FDA website (www.fda.gov), both text and graphics, are not copyrighted. They are in the public domain and may be republished, reprinted and otherwise used freely by anyone without the need to obtain permission from FDA. Credit to the U.S. Food and Drug Administration as the source is appreciated but not required. | |
Retrosynthesis Analysis
AI-Powered Synthesis Planning: Our tool employs the Template_relevance Pistachio, Template_relevance Bkms_metabolic, Template_relevance Pistachio_ringbreaker, Template_relevance Reaxys, Template_relevance Reaxys_biocatalysis model, leveraging a vast database of chemical reactions to predict feasible synthetic routes.
One-Step Synthesis Focus: Specifically designed for one-step synthesis, it provides concise and direct routes for your target compounds, streamlining the synthesis process.
Accurate Predictions: Utilizing the extensive PISTACHIO, BKMS_METABOLIC, PISTACHIO_RINGBREAKER, REAXYS, REAXYS_BIOCATALYSIS database, our tool offers high-accuracy predictions, reflecting the latest in chemical research and data.
Strategy Settings
Precursor scoring | Relevance Heuristic |
---|---|
Min. plausibility | 0.01 |
Model | Template_relevance |
Template Set | Pistachio/Bkms_metabolic/Pistachio_ringbreaker/Reaxys/Reaxys_biocatalysis |
Top-N result to add to graph | 6 |
Feasible Synthetic Routes
試験管内研究製品の免責事項と情報
BenchChemで提示されるすべての記事および製品情報は、情報提供を目的としています。BenchChemで購入可能な製品は、生体外研究のために特別に設計されています。生体外研究は、ラテン語の "in glass" に由来し、生物体の外で行われる実験を指します。これらの製品は医薬品または薬として分類されておらず、FDAから任何の医療状態、病気、または疾患の予防、治療、または治癒のために承認されていません。これらの製品を人間または動物に体内に導入する形態は、法律により厳格に禁止されています。これらのガイドラインに従うことは、研究と実験において法的および倫理的な基準の遵守を確実にするために重要です。