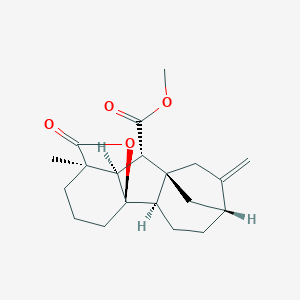
Gibberellin A9 methyl ester
概要
説明
Gibberellin A9 methyl ester is a pentacyclic diterpenoid compound that plays a significant role in plant growth and development. It is a methyl ester derivative of gibberellin A9, a type of gibberellin, which is a class of plant hormones known for promoting growth and elongation of cells in plants . Gibberellins were initially identified in the fungus Gibberella fujikuroi and have since been found in various plant species .
準備方法
Synthetic Routes and Reaction Conditions: Gibberellin A9 methyl ester can be synthesized from gibberellic acid through a series of chemical reactions. One common method involves converting methyl gibberellate into the methyl ester of 3-epigibberellin A1, followed by chlorination using triphenylphosphine and carbon tetrachloride. The resulting chlorides are then subjected to hydrogenolysis with tributyltin hydride to yield this compound .
Industrial Production Methods: Industrial production of this compound typically involves large-scale synthesis using similar chemical routes as described above. The process is optimized for yield and purity, ensuring that the final product meets the required standards for use in various applications .
化学反応の分析
Condensation Reactions for Heterocycle Formation
E3DAB undergoes condensation with aldehydes to form benzimidazole derivatives, a reaction leveraged in medicinal chemistry. For example:
-
Reaction with 4-methoxybenzaldehyde in dimethylformamide (DMF) under acidic conditions yields ethyl 2-(4-methoxyphenyl)-1H-benzo[d]imidazole-6-carboxylate via cyclocondensation .
-
Mechanism : The amino groups react with the aldehyde to form an imine intermediate, followed by cyclization to generate the benzimidazole core.
Key Conditions :
Reagent | Solvent | Temperature | Catalyst | Product Yield |
---|---|---|---|---|
4-Methoxybenzaldehyde | DMF | 100°C | Na₂S₂O₅ | 85–90% |
Polymerization via Polycondensation
E3DAB serves as a monomer in synthesizing polyamides and polybenzimidazoles:
-
Polyamide Synthesis : Direct polycondensation with aromatic dicarboxylic acids (e.g., terephthalic acid) using triphenyl phosphite (TPP) and pyridine produces thermally stable polyamides .
-
Reaction Steps :
-
Activation of carboxylic acids with TPP.
-
Formation of amide bonds between E3DAB’s amino groups and activated acids.
-
Polymer Properties :
Property | Value (Polyamide) | Notes |
---|---|---|
Thermal Stability | Up to 300°C | Decomposition onset in TGA |
Solubility | DMSO, DMF, NMP | Flexible film formation |
Ester Hydrolysis to Carboxylic Acid
The ethyl ester group in E3DAB undergoes hydrolysis under basic conditions:
-
Reaction : Treatment with aqueous NaOH (4 M) in ethanol at 90°C converts the ester to 3,5-diaminobenzoic acid .
-
Applications : The resulting diaminobenzoic acid is a precursor for metal-organic frameworks (MOFs) and covalent organic frameworks (COFs).
Optimized Hydrolysis Parameters :
Parameter | Optimal Value |
---|---|
NaOH Concentration | 4 M |
Temperature | 90°C |
Reaction Time | 12–24 hours |
Schiff Base Formation
The amino groups react with carbonyl compounds (aldehydes/ketones) to form Schiff bases:
-
Example : Reaction with cinnamaldehyde in ethanol produces a cinnamaldehyde-Schiff base conjugate, which exhibits UV absorption due to the extended π-system .
Characterization Data :
-
UV-Vis : λ<sub>max</sub> = 320–350 nm (conjugation-dependent) .
-
IR : N–H stretch (3350 cm⁻¹) replaced by C=N stretch (1630 cm⁻¹) post-reaction .
Acylation Reactions
E3DAB’s amino groups undergo acylation with acyl chlorides or anhydrides:
-
Synthesis of Cinnamide Derivatives : Treatment with cinnamoyl chloride in dichloromethane (DCM) yields 2′-(cinnamide)ethyl-3,5-diaminobenzoate, a photoactive monomer .
Reaction Efficiency :
Acylating Agent | Solvent | Reaction Time | Yield |
---|---|---|---|
Cinnamoyl Chloride | DCM | 4 hours | 78% |
Coordination with Metal Ions
E3DAB acts as a ligand for transition metals due to its amino and ester functionalities:
-
Complexation with Cu(II) : Forms stable complexes in aqueous ethanol, characterized by ESR and UV-Vis spectroscopy .
Stability Constants :
Metal Ion | log K (Stability Constant) |
---|---|
Cu(II) | 12.4 ± 0.3 |
Critical Analysis of Reaction Pathways
-
Steric Effects : The meta-substituted amino groups in E3DAB reduce steric hindrance compared to ortho-substituted analogs, enhancing reactivity in polycondensation .
-
Electronic Effects : Electron-donating amino groups activate the benzene ring for electrophilic substitution, though this is less common due to competing amine reactions.
科学的研究の応用
Seed Germination and Dormancy Regulation
GA9 Me is well-documented for its ability to promote seed germination by breaking dormancy in various plant species. Research indicates that it effectively enhances germination rates in crops such as:
- Lettuce
- Tomato
- Barley
Studies have shown that GA9 Me can lead to faster and more uniform germination by overcoming dormancy mechanisms in these species .
Stem Elongation
One of the primary applications of GA9 Me is in promoting stem elongation. This compound stimulates cell division and elongation, resulting in increased plant height. This characteristic is particularly beneficial for:
- Dwarf plant mutants : Researchers utilize GA9 Me to study the genetic basis of dwarfism and its potential reversibility.
- Agricultural practices : Manipulating plant architecture through GA9 Me can optimize crop yield and improve light interception .
Flowering Regulation
GA9 Me also plays a significant role in regulating flowering times, particularly in long-day plants. It can induce flowering or overcome photoperiod requirements, facilitating studies on:
- Bolting : The process where plants transition from vegetative to reproductive growth.
- Hormonal interplay : Understanding how gibberellins interact with other phytohormones to regulate flowering .
Fruit Development
In fruit crops, GA9 Me enhances fruit set and development. It promotes parthenocarpy (fruit development without fertilization) and improves fruit size and quality. Notable findings include:
- Increased fruit size in certain species.
- Enhanced overall crop yield through better fruit development strategies .
Modulation of NF-κB Pathway
Recent research highlights the potential of GA9 Me in modulating the NF-κB signaling pathway, which is implicated in various diseases, including cancer and inflammatory disorders. Applications include:
- Therapeutic uses : GA9 Me may serve as a probe for studying NF-κB activity or as a therapeutic agent for conditions associated with aberrant NF-κB signaling.
- Combination therapies : It can be combined with traditional chemotherapeutic agents to enhance treatment efficacy against proliferative diseases .
Case Study 1: Seed Germination Enhancement
A study demonstrated that applying GA9 Me at concentrations as low as significantly improved antheridium formation while inhibiting archegonium formation in Lygodium japonicum, showcasing its regulatory role in reproductive organ formation .
Case Study 2: Stem Growth Promotion
In rice cultivation, researchers found that GA9 Me application resulted in increased stem length and grain yield, underscoring its effectiveness as a growth promoter .
作用機序
Gibberellin A9 methyl ester exerts its effects by interacting with specific receptors in plant cells, leading to the activation of various signaling pathways. These pathways regulate gene expression and promote cell elongation, division, and differentiation. The primary molecular targets include DELLA proteins, which act as repressors of gibberellin signaling. Gibberellin binding to its receptor leads to the degradation of DELLA proteins, thereby activating downstream signaling events .
類似化合物との比較
- Gibberellin A1
- Gibberellin A3 (Gibberellic Acid)
- Gibberellin A4
- Gibberellin A7
Comparison: Gibberellin A9 methyl ester is unique due to its specific structural features and biological activity. Unlike gibberellin A1 and gibberellin A3, which have hydroxyl groups at specific positions, gibberellin A9 lacks these groups, resulting in different biological effects. This compound is particularly effective in promoting cell elongation and growth in certain plant species .
生物活性
Gibberellin A9 methyl ester (GA9-me) is a significant compound in the gibberellin family, which are plant hormones crucial for various growth and developmental processes. This article delves into the biological activity of GA9-me, exploring its mechanisms, effects on plant physiology, and applications in research and agriculture.
Overview of this compound
GA9-me is a methyl ester derivative of gibberellin A9, characterized by its pentacyclic diterpenoid structure. The compound plays a vital role in promoting plant growth, influencing processes such as seed germination, stem elongation, and flowering timing. Its chemical formula is with a molecular weight of 330.42 g/mol.
GA9-me functions primarily through its interaction with specific receptors in plant cells, triggering signaling cascades that regulate gene expression related to growth processes. Key aspects include:
- Target Sites : Primarily affects reproductive organs in certain plants, inducing antheridium formation while inhibiting archegonium formation .
- Biochemical Pathways : Involved in the gibberellin metabolic pathway, influencing various cellular processes through cell signaling and gene regulation .
- Environmental Influences : Its activity can be modulated by environmental factors such as light, temperature, and water availability.
Biological Activities
GA9-me exhibits various biological activities that are essential for plant development:
- Seed Germination : It effectively breaks dormancy in seeds of species like lettuce, tomato, and barley, leading to faster and more uniform germination.
- Stem Elongation : Promotes cell division and elongation, significantly increasing plant height. This property is particularly useful in manipulating plant architecture in agricultural settings.
- Flowering Regulation : Induces flowering in long-day plants and helps overcome photoperiod requirements for flowering .
- Fruit Development : Enhances fruit set and quality by promoting parthenocarpy (fruit development without fertilization) in certain crops.
Case Studies
- Germination Studies :
-
Stem Growth in Rice :
- A study involving rice plants showed that GA9-me application led to increased stem length and grain yield. This highlights its potential for enhancing crop productivity under controlled conditions.
- Reproductive Organ Formation :
Comparison with Other Gibberellins
The following table compares GA9-me with other gibberellins regarding their structural features and biological activities:
Compound Name | Molecular Formula | Unique Features |
---|---|---|
Gibberellic Acid | Precursor to many gibberellins; more hydroxyl groups | |
Gibberellin A2 | Distinct structural variations affecting activity | |
Gibberellin A3 | Known for its role in seed germination | |
Gibberellin A20 | Exhibits different biological activities |
GA9-me is unique due to its specific methylation at the carboxyl group, enhancing its stability and biological activity compared to other gibberellins .
特性
IUPAC Name |
methyl (1R,2R,5R,8R,9S,10R,11R)-11-methyl-6-methylidene-16-oxo-15-oxapentacyclo[9.3.2.15,8.01,10.02,8]heptadecane-9-carboxylate | |
---|---|---|
Source | PubChem | |
URL | https://pubchem.ncbi.nlm.nih.gov | |
Description | Data deposited in or computed by PubChem | |
InChI |
InChI=1S/C20H26O4/c1-11-9-19-10-12(11)5-6-13(19)20-8-4-7-18(2,17(22)24-20)15(20)14(19)16(21)23-3/h12-15H,1,4-10H2,2-3H3/t12-,13-,14-,15-,18-,19+,20-/m1/s1 | |
Source | PubChem | |
URL | https://pubchem.ncbi.nlm.nih.gov | |
Description | Data deposited in or computed by PubChem | |
InChI Key |
GKRMJALKMNRHGF-WARWBDHMSA-N | |
Source | PubChem | |
URL | https://pubchem.ncbi.nlm.nih.gov | |
Description | Data deposited in or computed by PubChem | |
Canonical SMILES |
CC12CCCC3(C1C(C45C3CCC(C4)C(=C)C5)C(=O)OC)OC2=O | |
Source | PubChem | |
URL | https://pubchem.ncbi.nlm.nih.gov | |
Description | Data deposited in or computed by PubChem | |
Isomeric SMILES |
C[C@@]12CCC[C@@]3([C@@H]1[C@@H]([C@]45[C@H]3CC[C@H](C4)C(=C)C5)C(=O)OC)OC2=O | |
Source | PubChem | |
URL | https://pubchem.ncbi.nlm.nih.gov | |
Description | Data deposited in or computed by PubChem | |
Molecular Formula |
C20H26O4 | |
Source | PubChem | |
URL | https://pubchem.ncbi.nlm.nih.gov | |
Description | Data deposited in or computed by PubChem | |
DSSTOX Substance ID |
DTXSID30555159 | |
Record name | Methyl (4aR,4bR,7R,9aR,10S,10aR)-1-methyl-8-methylidene-13-oxododecahydro-4a,1-(epoxymethano)-7,9a-methanobenzo[a]azulene-10-carboxylate | |
Source | EPA DSSTox | |
URL | https://comptox.epa.gov/dashboard/DTXSID30555159 | |
Description | DSSTox provides a high quality public chemistry resource for supporting improved predictive toxicology. | |
Molecular Weight |
330.4 g/mol | |
Source | PubChem | |
URL | https://pubchem.ncbi.nlm.nih.gov | |
Description | Data deposited in or computed by PubChem | |
CAS No. |
2112-08-5 | |
Record name | Methyl (4aR,4bR,7R,9aR,10S,10aR)-1-methyl-8-methylidene-13-oxododecahydro-4a,1-(epoxymethano)-7,9a-methanobenzo[a]azulene-10-carboxylate | |
Source | EPA DSSTox | |
URL | https://comptox.epa.gov/dashboard/DTXSID30555159 | |
Description | DSSTox provides a high quality public chemistry resource for supporting improved predictive toxicology. | |
Retrosynthesis Analysis
AI-Powered Synthesis Planning: Our tool employs the Template_relevance Pistachio, Template_relevance Bkms_metabolic, Template_relevance Pistachio_ringbreaker, Template_relevance Reaxys, Template_relevance Reaxys_biocatalysis model, leveraging a vast database of chemical reactions to predict feasible synthetic routes.
One-Step Synthesis Focus: Specifically designed for one-step synthesis, it provides concise and direct routes for your target compounds, streamlining the synthesis process.
Accurate Predictions: Utilizing the extensive PISTACHIO, BKMS_METABOLIC, PISTACHIO_RINGBREAKER, REAXYS, REAXYS_BIOCATALYSIS database, our tool offers high-accuracy predictions, reflecting the latest in chemical research and data.
Strategy Settings
Precursor scoring | Relevance Heuristic |
---|---|
Min. plausibility | 0.01 |
Model | Template_relevance |
Template Set | Pistachio/Bkms_metabolic/Pistachio_ringbreaker/Reaxys/Reaxys_biocatalysis |
Top-N result to add to graph | 6 |
Feasible Synthetic Routes
Q1: What is the stereochemical outcome of hydration reactions at the C-16 position in gibberellins like gibberellin A9?
A1: Research using spectroscopic analysis and X-ray crystallography has shown that the hydration of the 16-ene in gibberellins, including gibberellin A9, results in the formation of alcohols with a (16R)-stereochemistry. [, ] This stereospecificity is important for understanding the biological activity of these compounds.
Q2: Can gibberellin A9 methyl ester be synthesized from other gibberellins, and what does this tell us about its structure?
A2: Yes, this compound can be synthesized from both gibberellic acid and a mixture of gibberellins A4/A7. [] This synthesis confirms the structural similarities between these gibberellins and provides insights into the potential biosynthetic pathways of this compound in plants.
Q3: How is this compound metabolized by fungi, and what are the implications for its biological activity?
A3: this compound is metabolized by certain fungi, such as Gibberella fujikuroi and Rhizopus nigricans. [] The specific metabolites produced vary depending on the fungal species. For example, Gibberella fujikuroi converts this compound primarily into gibberellins A20 and A40, along with other metabolites like didehydrogibberellin A9 derivatives and hydroxylated forms. This metabolism highlights the potential for biotransformation of this compound in the environment and suggests possible roles for these metabolites in plant-fungal interactions.
Q4: Has this compound been identified in any natural sources?
A4: Yes, this compound has been identified as a naturally occurring substance in the plant Lygodium japonicum where it plays a role in regulating the formation of reproductive organs. [] This discovery suggests a potential ecological role for this compound and highlights the diversity of plant hormones in nature.
Q5: Does this compound exhibit estrogenic activity?
A5: this compound, along with other gibberellins (GA9, GA4, GA3, and their methyl esters), were tested for estrogenic activity using a recombinant yeast bioassay and were found to have no detectable activity. [] This finding suggests that this compound does not directly interact with the human estrogen receptor.
試験管内研究製品の免責事項と情報
BenchChemで提示されるすべての記事および製品情報は、情報提供を目的としています。BenchChemで購入可能な製品は、生体外研究のために特別に設計されています。生体外研究は、ラテン語の "in glass" に由来し、生物体の外で行われる実験を指します。これらの製品は医薬品または薬として分類されておらず、FDAから任何の医療状態、病気、または疾患の予防、治療、または治癒のために承認されていません。これらの製品を人間または動物に体内に導入する形態は、法律により厳格に禁止されています。これらのガイドラインに従うことは、研究と実験において法的および倫理的な基準の遵守を確実にするために重要です。