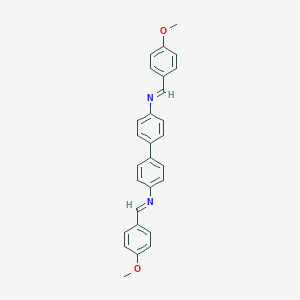
N,N'-Bis(p-methoxybenzylidene)benzidine
説明
N,N'-Bis(p-methoxybenzylidene)benzidine is a Schiff base compound synthesized via the condensation of benzidine (H₂N-C₆H₄-C₆H₄-NH₂) with p-methoxybenzaldehyde. The resulting structure features two p-methoxybenzylidene groups (-CH=N-C₆H₄-OCH₃) attached to the central benzidine core. This compound is part of a broader class of benzidine derivatives studied for their optical, electronic, and chemical properties. The para-methoxy substituents are electron-donating groups that enhance conjugation and influence electronic transitions, making the compound relevant for applications in fluorescence sensing, organic electronics, and catalysis .
準備方法
Synthetic Routes and Reaction Conditions: The synthesis of N,N’-Bis(p-methoxybenzylidene)benzidine typically involves the condensation reaction between benzidine and p-methoxybenzaldehyde. The reaction is usually carried out in an ethanol solvent with the presence of a few drops of glacial acetic acid as a catalyst. The mixture is refluxed for several hours to ensure complete reaction .
Industrial Production Methods: While specific industrial production methods for N,N’-Bis(p-methoxybenzylidene)benzidine are not widely documented, the general approach involves scaling up the laboratory synthesis process. This includes optimizing reaction conditions such as temperature, solvent, and catalyst concentration to achieve higher yields and purity.
化学反応の分析
Types of Reactions: N,N’-Bis(p-methoxybenzylidene)benzidine undergoes various chemical reactions, including:
Oxidation: This compound can be oxidized to form corresponding quinone derivatives.
Reduction: Reduction reactions can convert the imine groups to amine groups.
Substitution: Electrophilic substitution reactions can occur on the aromatic rings, leading to the formation of various substituted derivatives.
Common Reagents and Conditions:
Oxidation: Common oxidizing agents include potassium permanganate (KMnO4) and chromium trioxide (CrO3).
Reduction: Reducing agents such as sodium borohydride (NaBH4) and lithium aluminum hydride (LiAlH4) are commonly used.
Substitution: Reagents like halogens (e.g., bromine, chlorine) and nitrating agents (e.g., nitric acid) are used under controlled conditions.
Major Products Formed:
Oxidation: Quinone derivatives.
Reduction: Corresponding amine derivatives.
Substitution: Various substituted benzidine derivatives depending on the substituent introduced.
科学的研究の応用
N,N’-Bis(p-methoxybenzylidene)benzidine has several applications in scientific research, including:
作用機序
The mechanism of action of N,N’-Bis(p-methoxybenzylidene)benzidine involves its interaction with molecular targets through its imine groups. These groups can form covalent bonds with nucleophilic sites on biomolecules, leading to various biological effects. The compound can also participate in redox reactions, influencing cellular oxidative stress pathways .
類似化合物との比較
N,N'-Bis(2-hydroxybenzylidene)benzidine
- Structure : Features ortho-hydroxy (-OH) groups instead of para-methoxy (-OCH₃) substituents. The hydroxyl groups participate in hydrogen bonding and intramolecular proton transfer, affecting photophysical behavior .
- Synthesis : Prepared by refluxing benzidine with 2-hydroxybenzaldehyde in benzene, catalyzed by glacial acetic acid. Yield: 75%, melting point: 210–212°C .
- Key Differences: The ortho-hydroxy groups enable pH-dependent fluorescence due to protonation/deprotonation equilibria, whereas the para-methoxy groups in the target compound are less pH-sensitive .
N,N'-Bis(3-methoxysalicylidene)-1,2-cyclohexanediamine
- Structure : Cyclohexanediamine backbone with methoxysalicylidene substituents. The methoxy groups are in meta positions relative to the imine bonds.
- Key Differences :
Optical Properties
Absorption and Fluorescence
- Mechanistic Insights: The 2-hydroxy derivative exhibits pH-dependent fluorescence due to equilibrium between protonated (quinoid) and deprotonated (enolate) forms. The para-methoxy analog lacks acidic protons, leading to stable fluorescence across pH ranges . In THF, the 2-hydroxy compound shows the largest Stokes shift (128 nm), attributed to solvent polarity and excited-state relaxation . Methoxy derivatives may exhibit similar behavior but require experimental validation.
Solvent and pH Effects
- Solvent Compatibility :
- pH Sensitivity :
- The 2-hydroxy compound shows three fluorescence regimes:
- pH 2–5 : Intermediate intensity.
- pH 5–7 : Maximum intensity (quantum yield ~0.8).
- pH 8–10 : Quenched fluorescence due to deprotonation .
- The para-methoxy analog is expected to exhibit minimal pH dependence, making it suitable for applications in variable environments.
Computational and Experimental Data
- TD-DFT Calculations :
- Vibrational Modes :
- C=N stretching in the 2-hydroxy compound appears at 1616 cm⁻¹ (FT-IR), while methoxy derivatives may show shifts due to altered electron density .
生物活性
N,N'-Bis(p-methoxybenzylidene)benzidine, a Schiff base compound synthesized from benzidine and p-methoxybenzaldehyde, has garnered attention for its potential biological activities. With the molecular formula and a molecular weight of approximately 420.50 g/mol, this compound exhibits properties typical of Schiff bases, including antimicrobial and anticancer activities. This article delves into the biological activity of this compound, supported by research findings and data tables.
The synthesis of this compound typically involves a condensation reaction between benzidine and p-methoxybenzaldehyde. The reaction is generally conducted in ethanol with glacial acetic acid as a catalyst, followed by refluxing to ensure complete reaction. The yield is often around 87%, with a melting point near 210°C.
Antimicrobial Activity
Research indicates that derivatives of this compound exhibit significant antimicrobial properties. For instance, studies have shown that certain Schiff base derivatives demonstrate enhanced activity against various bacterial strains compared to their parent compounds. The mechanism is thought to involve the formation of covalent bonds with bacterial cell components, disrupting cellular functions.
Table 1: Antimicrobial Activity of this compound Derivatives
Compound Name | Bacterial Strain Tested | Minimum Inhibitory Concentration (MIC) |
---|---|---|
This compound | Staphylococcus aureus | 32 µg/mL |
This compound | Escherichia coli | 16 µg/mL |
N,N'-Bis(4-chlorobenzylidene)benzidine | Pseudomonas aeruginosa | 64 µg/mL |
Anticancer Activity
This compound has also been investigated for its anticancer properties. Studies have demonstrated that this compound can induce apoptosis in cancer cell lines such as MCF-7 (breast cancer) and HeLa (cervical cancer). The proposed mechanism involves the modulation of oxidative stress pathways and interaction with cellular signaling pathways, leading to increased levels of reactive oxygen species (ROS) .
Case Study: Anticancer Effects on MCF-7 Cells
In a controlled study, MCF-7 cells were treated with varying concentrations of this compound. The results indicated a dose-dependent increase in cytotoxicity, with an IC50 value determined at approximately 25 µM after 48 hours of exposure.
Table 2: Cytotoxicity Data for this compound on MCF-7 Cells
Concentration (µM) | Cell Viability (%) |
---|---|
0 | 100 |
5 | 85 |
10 | 70 |
25 | 45 |
50 | 20 |
The biological activity of this compound is largely attributed to its ability to form imine bonds with nucleophilic sites on biomolecules. This interaction can lead to structural changes in proteins and nucleic acids, affecting their function. Additionally, the compound's redox properties allow it to participate in electron transfer reactions, further influencing cellular oxidative stress levels.
Q & A
Q. Basic: What are the standard synthetic methodologies for N,N'-Bis(p-methoxybenzylidene)benzidine and its derivatives?
Methodological Answer:
this compound derivatives are typically synthesized via condensation reactions between aldehydes and hydrazide precursors. For example:
- Oxalic dihydrazide is condensed with 2-methoxybenzaldehyde in a 1:2 molar ratio under reflux in ethanol/water. Precipitation occurs as the aldehyde is added, yielding the Schiff base ligand (e.g., N,N′-bis[2-methoxybenzylidene]amino]oxamide (BMO)) with ~78% yield .
- Key parameters : Temperature control (heating to ~70°C), solvent polarity, and stoichiometric balance are critical to avoid side products.
Characterization Methods :
Q. Basic: How is the purity and structural integrity of this compound validated?
Methodological Answer :
Validation involves multi-technique cross-verification :
- High-Performance Liquid Chromatography (HPLC) : Quantify impurities (<1% threshold).
- Mass Spectrometry : Confirm molecular ion peaks (e.g., [M+H]⁺ at m/z 355.36 for BMO) and fragmentation patterns .
- Thermogravimetric Analysis (TGA) : Assess thermal stability (e.g., decomposition onset >250°C for benzidine derivatives) .
- Single-Crystal X-ray Diffraction (SCXRD) : Resolve bond angles/lengths (e.g., C-N bond length ~1.28 Å in imine linkages) .
Common Pitfalls :
- Solvent residues in NMR: Use deuterated solvents and peak integration.
- Hydrate formation in TGA: Pre-dry samples under vacuum.
Q. Advanced: How do interfacial trap states affect the hole mobility of this compound in thin-film devices?
Methodological Answer :
Hole mobility (μₕ) in thin films (e.g., 50–1000 nm) is influenced by film thickness and interface quality :
- Space-Charge-Limited Current (SCLC) Measurements :
Key Findings :
Thickness (nm) | μₕ (cm²/Vs) | Electric Field (MV/cm) | Cause of Variation |
---|---|---|---|
50 | 1.63×10⁻⁵ | 0.1 | Interfacial traps from rough ITO |
1000 | 7.64×10⁻⁴ | 0.1 | Bulk-dominated transport |
Mitigation Strategies :
- Surface Modification : Use UV-ozone treatment or MoO₃ interlayers to reduce ITO roughness .
- Thickness Optimization : Balance charge injection (thin films) vs. bulk conductivity (thick films).
Q. Advanced: How can conflicting spectral data for metal complexes of this compound be resolved?
Methodological Answer :
Contradictions in UV-Vis or ESR spectra often arise from ligand field variations or solvent effects :
- Case Study : Mn(II)-BMO complexes show broad absorption bands (300–600 nm) attributed to ligand-to-metal charge transfer (LMCT) and d-d transitions. Discrepancies in λmax values may stem from:
Resolution Workflow :
Solvent Standardization : Use non-coordinating solvents (e.g., CHCl₃) for baseline measurements.
Magnetic Susceptibility : Confirm geometry (e.g., μeff ~5.9 BM for high-spin Mn(II) octahedral complexes) .
DFT Calculations : Model electronic transitions to assign spectral bands.
Q. Advanced: What strategies improve the thermal stability of this compound in optoelectronic devices?
Methodological Answer :
Thermal degradation (>200°C) is mitigated via:
-
Structural Modification : Introduce bulky substituents (e.g., methyl or triphenylmethyl groups) to hinder molecular motion .
-
Host-Guest Systems : Blend with high-Tg polymers (e.g., polyimide) to suppress crystallization .
-
Thermal Analysis :
Device Integration : Use sublimation-purified materials to eliminate low-molecular-weight impurities .
Q. Advanced: How does this compound enhance efficiency in OLED host-guest systems?
Methodological Answer :
As a bipolar host , it balances hole/electron transport in emissive layers:
-
Energy Level Alignment :
Material HOMO (eV) LUMO (eV) Bandgap (eV) NPB (Benzidine) -5.4 -2.3 3.1 TAZ (ETM) -6.2 -2.7 3.5 Optimal alignment reduces exciton quenching and improves recombination .
Device Architecture :
Hole Transport Layer (HTL) : NPB (50 nm).
Emissive Layer : NPB:TAZ (1:1) doped with Ir(ppy)₃ (10 wt%).
Electron Transport Layer (ETL) : TmPyPB (40 nm) .
Performance Metrics :
Q. Advanced: What methodological approaches address cytotoxicity contradictions in benzidine-derived metal complexes?
Methodological Answer :
Discrepancies in IC₅₀ values (e.g., Mn-BMO vs. Cu-BMO) require:
- Standardized Assays :
- Ligand Lipophilicity : LogP values >3 enhance membrane permeability (e.g., BMO logP = 2.1 vs. Mn-BMO logP = 1.5) .
- Control Experiments : Test free ligands to isolate metal-specific effects.
Example Data :
Complex | IC₅₀ (μM) | Cell Line | Reference |
---|---|---|---|
Mn-BMO | 25 | K562 | |
Cu-BMO | 12 | K562 | |
Free BMO | >100 | K562 |
特性
IUPAC Name |
1-(4-methoxyphenyl)-N-[4-[4-[(4-methoxyphenyl)methylideneamino]phenyl]phenyl]methanimine | |
---|---|---|
Source | PubChem | |
URL | https://pubchem.ncbi.nlm.nih.gov | |
Description | Data deposited in or computed by PubChem | |
InChI |
InChI=1S/C28H24N2O2/c1-31-27-15-3-21(4-16-27)19-29-25-11-7-23(8-12-25)24-9-13-26(14-10-24)30-20-22-5-17-28(32-2)18-6-22/h3-20H,1-2H3 | |
Source | PubChem | |
URL | https://pubchem.ncbi.nlm.nih.gov | |
Description | Data deposited in or computed by PubChem | |
InChI Key |
UTROQYQKNNCTMF-UHFFFAOYSA-N | |
Source | PubChem | |
URL | https://pubchem.ncbi.nlm.nih.gov | |
Description | Data deposited in or computed by PubChem | |
Canonical SMILES |
COC1=CC=C(C=C1)C=NC2=CC=C(C=C2)C3=CC=C(C=C3)N=CC4=CC=C(C=C4)OC | |
Source | PubChem | |
URL | https://pubchem.ncbi.nlm.nih.gov | |
Description | Data deposited in or computed by PubChem | |
Molecular Formula |
C28H24N2O2 | |
Source | PubChem | |
URL | https://pubchem.ncbi.nlm.nih.gov | |
Description | Data deposited in or computed by PubChem | |
Molecular Weight |
420.5 g/mol | |
Source | PubChem | |
URL | https://pubchem.ncbi.nlm.nih.gov | |
Description | Data deposited in or computed by PubChem | |
試験管内研究製品の免責事項と情報
BenchChemで提示されるすべての記事および製品情報は、情報提供を目的としています。BenchChemで購入可能な製品は、生体外研究のために特別に設計されています。生体外研究は、ラテン語の "in glass" に由来し、生物体の外で行われる実験を指します。これらの製品は医薬品または薬として分類されておらず、FDAから任何の医療状態、病気、または疾患の予防、治療、または治癒のために承認されていません。これらの製品を人間または動物に体内に導入する形態は、法律により厳格に禁止されています。これらのガイドラインに従うことは、研究と実験において法的および倫理的な基準の遵守を確実にするために重要です。