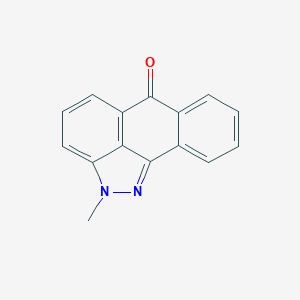
JNK阻害剤II、ネガティブコントロール
概要
説明
科学的研究の応用
Cell Signaling Studies
JNK Inhibitor II is extensively used in cell signaling research to elucidate the role of JNK in various biological processes. For instance, studies have shown that JNK activation plays a pivotal role in regulating NETosis—a form of cell death in neutrophils induced by Gram-negative bacteria such as E. coli and Pseudomonas aeruginosa. The use of JNK Inhibitor II demonstrated that inhibiting JNK activity significantly suppressed NETosis without inducing apoptosis .
Cancer Research
In cancer biology, JNK signaling has been implicated in oncogenic transformation and apoptosis. Research indicates that JNK inhibitors can modulate cell proliferation in cancer cells. For example, SP600125 has been shown to activate Müllerian Inhibiting Substance Receptor II-mediated signaling pathways in reproductive tract cancer cells, suggesting potential therapeutic applications .
Kidney Disease Models
Recent studies have explored the effects of JNK inhibition on renal progenitor cells, linking JNK signaling with self-renewal and protection against ferroptosis—a form of regulated cell death associated with kidney diseases. The application of JNK inhibitors like AEG3482 (related to JNK Inhibitor II) has shown decreased proliferation and altered gene expression in urine-derived renal progenitor cells, indicating its role in nephrogenesis .
Case Study 1: NETosis Regulation
A study investigated the role of JNK in regulating NETosis induced by LPS. Neutrophils treated with the JNK inhibitor showed a marked reduction in NET formation when stimulated with LPS, highlighting the importance of JNK signaling in immune responses .
Case Study 2: Ferroptosis in Kidney Cells
In a model using urine-derived renal progenitor cells, the application of a JNK inhibitor revealed insights into the mechanisms underlying ferroptosis and its implications for chronic kidney disease. The inhibition led to decreased oxidative phosphorylation and increased lipid peroxidation, suggesting that manipulating JNK pathways could offer new strategies for kidney regeneration therapies .
作用機序
Target of Action
The primary target of the JNK Inhibitor II, Negative Control is the c-Jun N-terminal kinases (JNKs), which are members of the mitogen-activated protein kinase (MAPK) family . JNKs regulate many physiological processes including inflammatory responses, cell proliferation, differentiation, survival, and death . The JNK family consists of three genes: JNK1, JNK2, and JNK3 . JNK1 and JNK2 are commonly expressed in many organs, while JNK3 is mainly expressed in cardiac smooth muscle, testis, and brain .
Mode of Action
The JNK Inhibitor II, Negative Control, also known as N¹-Methyl-1,9-pyrazoloanthrone, controls the biological activity of JNK . It inhibits JNK2 and JNK3 only at much higher concentrations (IC 50 = 18 µM and 24 µM, respectively) compared to SP600125 (IC 50 = 40 nM and 90 nM, respectively) . This inhibitor is primarily used for Phosphorylation & Dephosphorylation applications .
Biochemical Pathways
The activation of JNK requires dual phosphorylation at its Try and Thr residues, which is mediated by MKK4 and MKK7 . JNK pathway activation occurs in response to different stimuli during exposure of cell to biotic or abiotic stress like inflammation, oxidative stress, infection, osmotic stress, DNA damage or cytoskeletal changes . Different pathways are activated downstream of receptors such as transforming growth factor-β (TGF-β), G-protein coupled receptors (GPCRs), tumor necrosis factor (TNF) receptors, Wnt receptors, and Toll receptor complex . Also, JNK activation has been reported in response to endoplasmic reticulum stress (ER stress) .
Result of Action
The JNK Inhibitor II, Negative Control exhibits lower activity against JNK isoforms . It is a useful negative control for JNK Inhibitor II (SP600125) Jnk inhibitors have been shown to regulate many physiological processes, including inflammatory responses, cell proliferation, differentiation, survival, and death .
Action Environment
The action, efficacy, and stability of JNK Inhibitor II, Negative Control can be influenced by various environmental factors. For instance, JNK pathway activation occurs in response to different stimuli during exposure of cell to biotic or abiotic stress like inflammation, oxidative stress, infection, osmotic stress, DNA damage or cytoskeletal changes . Understanding these environmental influences is crucial for optimizing the use of this compound in research and potential therapeutic applications.
生化学分析
Biochemical Properties
JNK Inhibitor II, Negative Control, plays a crucial role in biochemical reactions by interacting with specific enzymes and proteins. It inhibits JNK2 and JNK3 only at much higher concentrations compared to SP600125, with IC50 values of 18 µM and 24 µM, respectively . This compound is primarily used to ensure the specificity of JNK inhibition in experimental setups. By serving as a negative control, it helps researchers distinguish between specific and non-specific effects of JNK inhibition. The interactions of JNK Inhibitor II, Negative Control, with JNK2 and JNK3 are characterized by competitive inhibition, where the compound competes with ATP for binding to the active site of the kinases .
Cellular Effects
JNK Inhibitor II, Negative Control, influences various cellular processes by modulating the activity of JNK signaling pathways. Although it has a lower affinity for JNK isoforms, it can still impact cell function at higher concentrations. The compound affects cell signaling pathways, gene expression, and cellular metabolism by inhibiting the phosphorylation of c-Jun, a critical transcription factor regulated by JNK . This inhibition can lead to altered expression of genes involved in cell proliferation, differentiation, and apoptosis. Additionally, JNK Inhibitor II, Negative Control, has been shown to reduce the expression of inflammatory cytokines such as interleukin-1 (IL-1) and tumor necrosis factor-alpha (TNF-α) in certain cell types .
Molecular Mechanism
The molecular mechanism of action of JNK Inhibitor II, Negative Control, involves competitive inhibition of JNK2 and JNK3. The compound binds to the ATP-binding site of these kinases, preventing ATP from binding and thereby inhibiting their catalytic activity . This inhibition leads to a decrease in the phosphorylation of downstream targets, such as c-Jun, which in turn affects gene expression and cellular responses. The specificity of JNK Inhibitor II, Negative Control, for JNK2 and JNK3 is lower than that of SP600125, making it a valuable tool for distinguishing specific JNK-mediated effects from non-specific ones in experimental studies .
Temporal Effects in Laboratory Settings
In laboratory settings, the effects of JNK Inhibitor II, Negative Control, can change over time due to its stability and degradation properties. The compound is stable when stored at -20°C and protected from light, with stock solutions remaining stable for up to three months . Over extended periods, the inhibitory effects of JNK Inhibitor II, Negative Control, may diminish due to degradation, which can impact the consistency of experimental results. Long-term studies have shown that the compound can maintain its inhibitory activity for several weeks, but its efficacy may decrease over time .
Dosage Effects in Animal Models
The effects of JNK Inhibitor II, Negative Control, vary with different dosages in animal models. At lower doses, the compound exhibits minimal inhibitory activity against JNK isoforms, making it an effective negative control. At higher doses, it can inhibit JNK2 and JNK3, leading to observable physiological effects . In animal studies, high doses of JNK Inhibitor II, Negative Control, have been associated with reduced tumor growth and decreased expression of pro-inflammatory cytokines . Toxic or adverse effects at high doses have not been extensively reported, but caution is advised when interpreting results from high-dose experiments.
Metabolic Pathways
JNK Inhibitor II, Negative Control, is involved in metabolic pathways related to the regulation of JNK signaling. The compound interacts with enzymes and cofactors that modulate the activity of JNK2 and JNK3, influencing metabolic flux and metabolite levels . By inhibiting JNK activity, JNK Inhibitor II, Negative Control, can affect the expression of genes involved in metabolic processes, such as glycolysis and oxidative phosphorylation . This modulation of metabolic pathways can have downstream effects on cellular energy production and overall metabolic homeostasis.
Transport and Distribution
The transport and distribution of JNK Inhibitor II, Negative Control, within cells and tissues are influenced by its chemical properties. The compound is cell-permeable, allowing it to enter cells and interact with intracellular targets . Once inside the cell, JNK Inhibitor II, Negative Control, can be distributed to various cellular compartments, including the cytoplasm and nucleus. The compound’s distribution is facilitated by its interaction with transporters and binding proteins that help localize it to specific cellular regions . This localization is crucial for its inhibitory effects on JNK signaling pathways.
Subcellular Localization
JNK Inhibitor II, Negative Control, exhibits specific subcellular localization patterns that influence its activity and function. The compound is primarily localized in the cytoplasm, where it interacts with JNK2 and JNK3 to inhibit their activity . Additionally, JNK Inhibitor II, Negative Control, can translocate to the nucleus, where it affects the phosphorylation of nuclear targets such as c-Jun . The subcellular localization of JNK Inhibitor II, Negative Control, is regulated by targeting signals and post-translational modifications that direct it to specific compartments or organelles .
準備方法
Synthetic Routes and Reaction Conditions
The synthesis of JNK Inhibitor II, Negative Control involves the methylation of SP600125, a known JNK inhibitor. The process typically includes the following steps:
Starting Material: SP600125.
Methylation: The methylation reaction is carried out using methyl iodide (CH₃I) in the presence of a base such as potassium carbonate (K₂CO₃) in an appropriate solvent like dimethyl sulfoxide (DMSO).
Purification: The product is purified using standard chromatographic techniques to obtain N¹-Methyl-1,9-pyrazoloanthrone with high purity.
Industrial Production Methods
While specific industrial production methods for JNK Inhibitor II, Negative Control are not widely documented, the general approach would involve scaling up the laboratory synthesis process. This includes optimizing reaction conditions, using industrial-grade reagents, and employing large-scale purification techniques to ensure consistent quality and yield.
化学反応の分析
Types of Reactions
JNK Inhibitor II, Negative Control primarily undergoes the following types of reactions:
Substitution Reactions: The methylation of SP600125 to form N¹-Methyl-1,9-pyrazoloanthrone is an example of a substitution reaction.
Oxidation and Reduction: While not commonly reported, potential oxidation or reduction reactions could modify the functional groups on the molecule.
Common Reagents and Conditions
Methylation: Methyl iodide (CH₃I) and potassium carbonate (K₂CO₃) in DMSO.
Oxidation/Reduction: Specific reagents would depend on the desired modification, such as hydrogen peroxide (H₂O₂) for oxidation or sodium borohydride (NaBH₄) for reduction.
Major Products
The primary product of interest is N¹-Methyl-1,9-pyrazoloanthrone, which is used as a negative control in JNK inhibition studies .
類似化合物との比較
Similar Compounds
SP600125: A potent JNK inhibitor with higher affinity for JNK isoforms.
JNK-IN-8: A covalent JNK inhibitor that suppresses tumor growth in triple-negative breast cancer.
BI-78D3: An inhibitor that blocks JNK activity by preventing protein-protein interactions
Uniqueness
JNK Inhibitor II, Negative Control is unique in its role as a negative control, providing a baseline to compare the effects of other JNK inhibitors. Its higher IC₅₀ values for JNK2 and JNK3 make it less effective as an inhibitor, but valuable for validating the specificity of JNK-targeted studies .
生物活性
JNK Inhibitor II, Negative Control (CAS 54642-23-8), is a small molecule primarily utilized in biological research to control the activity of c-Jun N-terminal kinase (JNK). It serves as a negative control for the more potent JNK inhibitor, SP600125. Understanding its biological activity is crucial for researchers studying signal transduction pathways, particularly those involving MAPK pathways.
- Chemical Formula : C₁₅H₁₀N₂O
- Molecular Weight : 234.2 g/mol
- Form : Yellow solid
- Purity : ≥97% by HPLC
- Cell Permeability : Yes
- Packaging : Packaged under inert gas to maintain stability and prevent degradation.
JNK Inhibitor II functions by inhibiting the phosphorylation activity of JNK, which is part of the MAPK signaling cascade. The compound is known to exhibit a competitive inhibition mechanism against ATP binding in the inactive state of the kinase (DFG-out conformation). This property allows it to serve as a control in experiments where JNK activity modulation is required.
Biological Activity and Applications
The biological activity of JNK Inhibitor II is characterized by its role as a negative control in various assays aimed at understanding JNK signaling pathways. Here are some key findings regarding its biological activity:
- Inhibition Profile :
- Research Applications :
Case Study 1: Schistosomiasis Research
A recent study focused on identifying potent schistosomicidal compounds involved screening for inhibitors targeting JNK pathways. The research highlighted that inhibiting JNK could affect parasite motility and survival. The study utilized JNK Inhibitor II as a control to compare the efficacy of newly identified compounds against Schistosoma mansoni .
Case Study 2: Cancer Research
In cancer biology, the modulation of JNK signaling has been linked to tumor progression and metastasis. Researchers have employed JNK Inhibitor II to delineate the role of JNK in macrophage polarization within tumor microenvironments. The inhibitor helped establish that altering JNK activity could shift macrophage responses from pro-inflammatory (M1) to anti-inflammatory (M2), influencing tumor growth dynamics .
Comparative Analysis of Inhibitors
Inhibitor | IC50 (µM) | Target Kinases | Mechanism |
---|---|---|---|
SP600125 | 40 (JNK1) | JNK1, JNK2, JNK3 | Competitive ATP binding |
JNK Inhibitor II | 18 (JNK2) | JNK2 | Competitive ATP binding |
Other Type II Inhibitors | Varies | Various PKs | DFG-out conformation binding |
特性
IUPAC Name |
14-methyl-14,15-diazatetracyclo[7.6.1.02,7.013,16]hexadeca-1(15),2,4,6,9(16),10,12-heptaen-8-one | |
---|---|---|
Source | PubChem | |
URL | https://pubchem.ncbi.nlm.nih.gov | |
Description | Data deposited in or computed by PubChem | |
InChI |
InChI=1S/C15H10N2O/c1-17-12-8-4-7-11-13(12)14(16-17)9-5-2-3-6-10(9)15(11)18/h2-8H,1H3 | |
Source | PubChem | |
URL | https://pubchem.ncbi.nlm.nih.gov | |
Description | Data deposited in or computed by PubChem | |
InChI Key |
ODZGYELAMAOARP-UHFFFAOYSA-N | |
Source | PubChem | |
URL | https://pubchem.ncbi.nlm.nih.gov | |
Description | Data deposited in or computed by PubChem | |
Canonical SMILES |
CN1C2=CC=CC3=C2C(=N1)C4=CC=CC=C4C3=O | |
Source | PubChem | |
URL | https://pubchem.ncbi.nlm.nih.gov | |
Description | Data deposited in or computed by PubChem | |
Molecular Formula |
C15H10N2O | |
Source | PubChem | |
URL | https://pubchem.ncbi.nlm.nih.gov | |
Description | Data deposited in or computed by PubChem | |
Molecular Weight |
234.25 g/mol | |
Source | PubChem | |
URL | https://pubchem.ncbi.nlm.nih.gov | |
Description | Data deposited in or computed by PubChem | |
Retrosynthesis Analysis
AI-Powered Synthesis Planning: Our tool employs the Template_relevance Pistachio, Template_relevance Bkms_metabolic, Template_relevance Pistachio_ringbreaker, Template_relevance Reaxys, Template_relevance Reaxys_biocatalysis model, leveraging a vast database of chemical reactions to predict feasible synthetic routes.
One-Step Synthesis Focus: Specifically designed for one-step synthesis, it provides concise and direct routes for your target compounds, streamlining the synthesis process.
Accurate Predictions: Utilizing the extensive PISTACHIO, BKMS_METABOLIC, PISTACHIO_RINGBREAKER, REAXYS, REAXYS_BIOCATALYSIS database, our tool offers high-accuracy predictions, reflecting the latest in chemical research and data.
Strategy Settings
Precursor scoring | Relevance Heuristic |
---|---|
Min. plausibility | 0.01 |
Model | Template_relevance |
Template Set | Pistachio/Bkms_metabolic/Pistachio_ringbreaker/Reaxys/Reaxys_biocatalysis |
Top-N result to add to graph | 6 |
Feasible Synthetic Routes
試験管内研究製品の免責事項と情報
BenchChemで提示されるすべての記事および製品情報は、情報提供を目的としています。BenchChemで購入可能な製品は、生体外研究のために特別に設計されています。生体外研究は、ラテン語の "in glass" に由来し、生物体の外で行われる実験を指します。これらの製品は医薬品または薬として分類されておらず、FDAから任何の医療状態、病気、または疾患の予防、治療、または治癒のために承認されていません。これらの製品を人間または動物に体内に導入する形態は、法律により厳格に禁止されています。これらのガイドラインに従うことは、研究と実験において法的および倫理的な基準の遵守を確実にするために重要です。