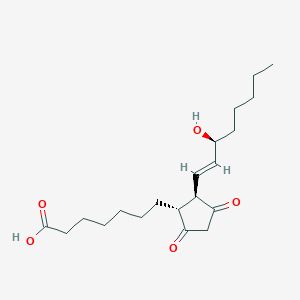
Prostaglandin K1
説明
Prostaglandin K1 (PGK1) is a 9,11-diketo analog of PGE1 or PGD1 . It is a PGE2 receptor subtype EP1 agonist that has 387-fold reduced potency compared to PGE2 . Prostaglandins are a group of physiologically active lipid compounds that have diverse hormone-like effects in animals .
Molecular Structure Analysis
Prostaglandins, including Prostaglandin K1, possess a unique and intricate molecular structure that underlies their diverse physiological functions . They have a distinctive twenty-carbon skeleton, featuring a central five-membered ring . This ring contains oxygen atoms at specific positions, imparting reactivity and distinct chemical properties to prostaglandins .
Chemical Reactions Analysis
Prostaglandins, including Prostaglandin K1, have unique functional groups attached to their hydrocarbon chains . These functional groups, such as hydroxyl (-OH), keto (=O), and carboxyl (-COOH) groups, contribute specific chemical properties to the prostaglandin, making it suitable for particular physiological functions .
科学的研究の応用
Prostanoid Receptors: Expression and Characterization
Prostaglandin signaling is crucial for a variety of biological processes, including blood pressure homeostasis, inflammation resolution, pain perception, and cell survival. Disruptions in prostanoid signaling are linked to numerous disease states .
Prostaglandin Receptors in Pulmonary Arterial Hypertension (PAH)
Prostaglandins and their receptors are significant in the development of PAH, influencing vasoconstriction, vascular smooth muscle cell proliferation, migration, inflammation, and extracellular matrix remodeling. Prostacyclin and related drugs are used clinically to treat PAH .
Prostaglandins in Tissue Differentiation and Regeneration
Prostaglandins play a role in the differentiation and tissue regeneration of various cell types and organs. Their involvement in motor neuron differentiation from neural stem cells is an area of ongoing research .
作用機序
Target of Action
Prostaglandin K1, like other prostaglandins, primarily targets G-protein-coupled receptors (GPCRs) . These receptors are specific to prostanoids and are associated with a variety of G-proteins .
Biochemical Pathways
Prostaglandins, including Prostaglandin K1, are derived enzymatically from fatty acids . They are involved in a wide range of roles from homeostasis of blood pressure and blood flow, the initiation and resolution of inflammation, the perception of pain, cell survival, and the progression of numerous disease states . These biomolecules act most often as autocrine or paracrine signaling agents and most have relatively short half-lives .
Pharmacokinetics
Prostaglandins in general have been shown to have relatively short half-lives . They are often administered via intra-arterial or intravenous infusions . The metabolism and pharmacokinetics of prostaglandins are complex and can be influenced by various factors, including the method of administration .
Action Environment
The action, efficacy, and stability of Prostaglandin K1 can be influenced by various environmental factors. For example, the abundance of aggressive bacteria was found to be positively correlated with the levels of certain prostaglandins . This suggests that the gut microbiota could potentially influence the action of Prostaglandin K1 .
Safety and Hazards
特性
IUPAC Name |
7-[(1R,2R)-2-[(E,3S)-3-hydroxyoct-1-enyl]-3,5-dioxocyclopentyl]heptanoic acid | |
---|---|---|
Source | PubChem | |
URL | https://pubchem.ncbi.nlm.nih.gov | |
Description | Data deposited in or computed by PubChem | |
InChI |
InChI=1S/C20H32O5/c1-2-3-6-9-15(21)12-13-17-16(18(22)14-19(17)23)10-7-4-5-8-11-20(24)25/h12-13,15-17,21H,2-11,14H2,1H3,(H,24,25)/b13-12+/t15-,16+,17+/m0/s1 | |
Source | PubChem | |
URL | https://pubchem.ncbi.nlm.nih.gov | |
Description | Data deposited in or computed by PubChem | |
InChI Key |
KJWZYMMLVHIVSU-IYCNHOCDSA-N | |
Source | PubChem | |
URL | https://pubchem.ncbi.nlm.nih.gov | |
Description | Data deposited in or computed by PubChem | |
Canonical SMILES |
CCCCCC(C=CC1C(C(=O)CC1=O)CCCCCCC(=O)O)O | |
Source | PubChem | |
URL | https://pubchem.ncbi.nlm.nih.gov | |
Description | Data deposited in or computed by PubChem | |
Isomeric SMILES |
CCCCC[C@@H](/C=C/[C@@H]1[C@H](C(=O)CC1=O)CCCCCCC(=O)O)O | |
Source | PubChem | |
URL | https://pubchem.ncbi.nlm.nih.gov | |
Description | Data deposited in or computed by PubChem | |
Molecular Formula |
C20H32O5 | |
Source | PubChem | |
URL | https://pubchem.ncbi.nlm.nih.gov | |
Description | Data deposited in or computed by PubChem | |
DSSTOX Substance ID |
DTXSID401348011 | |
Record name | Prostaglandin K1 | |
Source | EPA DSSTox | |
URL | https://comptox.epa.gov/dashboard/DTXSID401348011 | |
Description | DSSTox provides a high quality public chemistry resource for supporting improved predictive toxicology. | |
Molecular Weight |
352.5 g/mol | |
Source | PubChem | |
URL | https://pubchem.ncbi.nlm.nih.gov | |
Description | Data deposited in or computed by PubChem | |
CAS RN |
69413-73-6 | |
Record name | Prostaglandin K1 | |
Source | EPA DSSTox | |
URL | https://comptox.epa.gov/dashboard/DTXSID401348011 | |
Description | DSSTox provides a high quality public chemistry resource for supporting improved predictive toxicology. | |
Retrosynthesis Analysis
AI-Powered Synthesis Planning: Our tool employs the Template_relevance Pistachio, Template_relevance Bkms_metabolic, Template_relevance Pistachio_ringbreaker, Template_relevance Reaxys, Template_relevance Reaxys_biocatalysis model, leveraging a vast database of chemical reactions to predict feasible synthetic routes.
One-Step Synthesis Focus: Specifically designed for one-step synthesis, it provides concise and direct routes for your target compounds, streamlining the synthesis process.
Accurate Predictions: Utilizing the extensive PISTACHIO, BKMS_METABOLIC, PISTACHIO_RINGBREAKER, REAXYS, REAXYS_BIOCATALYSIS database, our tool offers high-accuracy predictions, reflecting the latest in chemical research and data.
Strategy Settings
Precursor scoring | Relevance Heuristic |
---|---|
Min. plausibility | 0.01 |
Model | Template_relevance |
Template Set | Pistachio/Bkms_metabolic/Pistachio_ringbreaker/Reaxys/Reaxys_biocatalysis |
Top-N result to add to graph | 6 |
Feasible Synthetic Routes
試験管内研究製品の免責事項と情報
BenchChemで提示されるすべての記事および製品情報は、情報提供を目的としています。BenchChemで購入可能な製品は、生体外研究のために特別に設計されています。生体外研究は、ラテン語の "in glass" に由来し、生物体の外で行われる実験を指します。これらの製品は医薬品または薬として分類されておらず、FDAから任何の医療状態、病気、または疾患の予防、治療、または治癒のために承認されていません。これらの製品を人間または動物に体内に導入する形態は、法律により厳格に禁止されています。これらのガイドラインに従うことは、研究と実験において法的および倫理的な基準の遵守を確実にするために重要です。