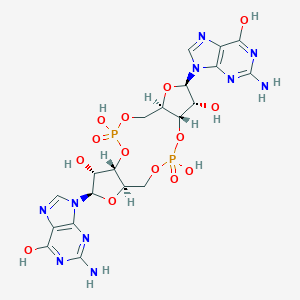
c-di-GMP
概要
説明
環状ビス-(3', 5')-二量体グアノシン一リン酸(二ナトリウム塩)は、1987年に発見されたヌクレオチドベースのシグナル伝達分子です。 これは、細菌における運動性、バイオフィルム形成、毒性、細胞周期進行など、さまざまな生物学的プロセスを調節する重要な役割を果たすセカンドメッセンジャーです 。 この化合物は、ヒト細胞の特定の受容体に結合し、宿主細胞の応答を調節し、治療標的としての可能性を示すことでも知られています .
科学的研究の応用
作用機序
環状ビス-(3', 5')-二量体グアノシン一リン酸(二ナトリウム塩)は、細菌および真核細胞の特定の受容体に結合することでその効果を発揮します。 細菌では、その細胞内濃度を制御するジグアニレートシクラーゼやホスホジエステラーゼと相互作用することで、さまざまな細胞活動を調節します 。 真核細胞では、STING(インターフェロン遺伝子の刺激因子)などの受容体に結合し、免疫応答を調節し、抗がん特性を示します .
生化学分析
Biochemical Properties
Cyclic Diguanylic Acid interacts with a wide range of enzymes, proteins, and other biomolecules. The intracellular concentration of Cyclic Diguanylic Acid is controlled by diguanylate cyclases (DGCs) and phosphodiesterases (PDEs) . DGCs catalyze Cyclic Diguanylic Acid production by consuming two GTP molecules, whereas PDEs catalyze the degradation of Cyclic Diguanylic Acid into a pGpG linear nucleotide or two GTP molecules . Signal transduction occurs through Cyclic Diguanylic Acid binding to downstream receptors, triggered by sensing environmental or cellular cues .
Cellular Effects
Cyclic Diguanylic Acid has a profound impact on various types of cells and cellular processes. It influences cell function by regulating cell motility, biofilm formation and dispersion, cell division, differentiation, quorum sensing, and virulence . It also controls transcription initiation and termination, translation, exopolysaccharide (EPS) synthesis and secretion, flagellar and type III secretion system assembly, cell cycle progression, proteolysis of target proteins, and involvement in the localization of target proteins .
Molecular Mechanism
Cyclic Diguanylic Acid exerts its effects at the molecular level through binding interactions with biomolecules, enzyme inhibition or activation, and changes in gene expression . It binds to a diverse array of protein or RNA effectors which include PilZ domain-containing proteins, transcription factors, proteases, kinases, and riboswitches .
Temporal Effects in Laboratory Settings
In laboratory settings, Cyclic Diguanylic Acid shows dynamic changes with high temporal resolution . A genetically encoded biosensor has been developed to track Cyclic Diguanylic Acid in single cells and with high temporal resolution over extended imaging times .
Dosage Effects in Animal Models
In animal models, the effects of Cyclic Diguanylic Acid vary with different dosages . It enhances protective innate immunity in a murine model of pertussis . The immune responses were associated with an earlier and more vigorous expression of Th1-type cytokines, as well as an increase in the induction of nitric oxide in the lungs of treated animals, resulting in significant reduction of bacterial numbers in the lungs of infected mice .
Metabolic Pathways
Cyclic Diguanylic Acid is involved in numerous metabolic pathways. It interacts with a multitude of enzymes and cofactors . Proteins involved in Cyclic Diguanylic Acid metabolism and regulation, such as diguanylate cyclases, phosphodiesterases, and PilZ-containing proteins, were encoded in bacterial genomes .
Transport and Distribution
Cyclic Diguanylic Acid is transported and distributed within cells and tissues . As Cyclic Diguanylic Acid is a small molecule which can diffuse easily inside the cell, there must be a mechanism for activating only specific Cyclic Diguanylic Acid-associated pathways at a given point of time while avoiding any undesirable crosstalk between the numerous Cyclic Diguanylic Acid-binding partners .
Subcellular Localization
It has been shown that the transcriptional regulator, VpsT, requires Cyclic Diguanylic Acid binding for subcellular localization and activity .
準備方法
合成経路と反応条件
環状ビス-(3', 5')-二量体グアノシン一リン酸(二ナトリウム塩)は、化学的および酵素的の両方の手法を用いて合成することができます。酵素合成は最も効率的な方法と考えられています。 これは、2つのグアノシン-5'-三リン酸分子を環状ビス-(3', 5')-二量体グアノシン一リン酸に変換する触媒作用を有するジグアニレートシクラーゼの使用を伴います 。 反応条件は通常、酵素活性を促進するためにマグネシウムまたはマンガンなどの二価カチオンの存在を必要とします .
工業生産方法
工業規模での生産では、その効率性とスケーラビリティのために酵素法が好まれます。より安定しており、生成物阻害を受けにくい好熱性ジグアニレートシクラーゼがしばしば使用されます。 これらの酵素は、少量の生体触媒を使用して、数百ミリグラムの環状ビス-(3', 5')-二量体グアノシン一リン酸を生成することができます .
化学反応の分析
反応の種類
環状ビス-(3', 5')-二量体グアノシン一リン酸(二ナトリウム塩)は、加水分解や特定の受容体との結合相互作用など、さまざまな化学反応を起こします。 環状ビス-(3', 5')-二量体グアノシン一リン酸の加水分解は、それを線状ヌクレオチドまたはグアノシン一リン酸に分解するホスホジエステラーゼによって触媒されます .
一般的な試薬と条件
加水分解反応は通常、EALまたはHD-GYPドメインを持つホスホジエステラーゼの存在を必要とします。 これらの酵素は、生理的条件下で環状ビス-(3', 5')-二量体グアノシン一リン酸の分解を促進します .
生成される主要な生成物
加水分解反応の主要な生成物は、5'-ホスホグアニリル-(3'→5')-グアノシンやグアノシン一リン酸などの線状ヌクレオチドです .
科学研究への応用
環状ビス-(3', 5')-二量体グアノシン一リン酸(二ナトリウム塩)は、幅広い科学研究への応用を有しています。
化学: 細菌のシグナル伝達経路の調節機構や、細胞プロセスにおけるセカンドメッセンジャーの役割を研究するために使用されます.
生物学: これは、細菌の運動性、バイオフィルム形成、および毒性を理解する上で重要な役割を果たします。
医学: 環状ビス-(3', 5')-二量体グアノシン一リン酸は、抗菌剤や免疫調節療法の開発のための潜在的な治療標的として研究されています.
類似化合物との比較
環状ビス-(3', 5')-二量体グアノシン一リン酸(二ナトリウム塩)は、細菌のシグナル伝達における特定の役割と、宿主の免疫応答を調節する能力のために、他のセカンドメッセンジャーとは異なります。類似の化合物には、次のようなものがあります。
環状アデノシン一リン酸(c-AMP): 原核生物と真核生物の両方でさまざまな細胞プロセスに関与する別のセカンドメッセンジャー.
環状グアノシン一リン酸(c-GMP): 血管のトーン、細胞の成長、およびアポトーシスを調節するシグナル伝達分子.
環状ジ-アデノシン一リン酸(c-di-AMP): 細菌の成長、細胞壁の維持、および毒性に関連するセカンドメッセンジャー.
環状ビス-(3', 5')-二量体グアノシン一リン酸(二ナトリウム塩)は、細菌のシグナル伝達と宿主の免疫調節の両方における二重の役割のために際立っており、治療介入のための有望な標的となっています .
特性
IUPAC Name |
2-amino-9-[(1S,6R,8R,9R,10S,15R,17R,18R)-17-(2-amino-6-oxo-1H-purin-9-yl)-3,9,12,18-tetrahydroxy-3,12-dioxo-2,4,7,11,13,16-hexaoxa-3λ5,12λ5-diphosphatricyclo[13.3.0.06,10]octadecan-8-yl]-1H-purin-6-one | |
---|---|---|
Source | PubChem | |
URL | https://pubchem.ncbi.nlm.nih.gov | |
Description | Data deposited in or computed by PubChem | |
InChI |
InChI=1S/C20H24N10O14P2/c21-19-25-13-7(15(33)27-19)23-3-29(13)17-9(31)11-5(41-17)1-39-45(35,36)44-12-6(2-40-46(37,38)43-11)42-18(10(12)32)30-4-24-8-14(30)26-20(22)28-16(8)34/h3-6,9-12,17-18,31-32H,1-2H2,(H,35,36)(H,37,38)(H3,21,25,27,33)(H3,22,26,28,34)/t5-,6-,9-,10-,11-,12-,17-,18-/m1/s1 | |
Source | PubChem | |
URL | https://pubchem.ncbi.nlm.nih.gov | |
Description | Data deposited in or computed by PubChem | |
InChI Key |
PKFDLKSEZWEFGL-MHARETSRSA-N | |
Source | PubChem | |
URL | https://pubchem.ncbi.nlm.nih.gov | |
Description | Data deposited in or computed by PubChem | |
Canonical SMILES |
C1C2C(C(C(O2)N3C=NC4=C3N=C(NC4=O)N)O)OP(=O)(OCC5C(C(C(O5)N6C=NC7=C6N=C(NC7=O)N)O)OP(=O)(O1)O)O | |
Source | PubChem | |
URL | https://pubchem.ncbi.nlm.nih.gov | |
Description | Data deposited in or computed by PubChem | |
Isomeric SMILES |
C1[C@@H]2[C@H]([C@H]([C@@H](O2)N3C=NC4=C3N=C(NC4=O)N)O)OP(=O)(OC[C@@H]5[C@H]([C@H]([C@@H](O5)N6C=NC7=C6N=C(NC7=O)N)O)OP(=O)(O1)O)O | |
Source | PubChem | |
URL | https://pubchem.ncbi.nlm.nih.gov | |
Description | Data deposited in or computed by PubChem | |
Molecular Formula |
C20H24N10O14P2 | |
Source | PubChem | |
URL | https://pubchem.ncbi.nlm.nih.gov | |
Description | Data deposited in or computed by PubChem | |
DSSTOX Substance ID |
DTXSID801027528 | |
Record name | Cyclic diguanylic acid | |
Source | EPA DSSTox | |
URL | https://comptox.epa.gov/dashboard/DTXSID801027528 | |
Description | DSSTox provides a high quality public chemistry resource for supporting improved predictive toxicology. | |
Molecular Weight |
690.4 g/mol | |
Source | PubChem | |
URL | https://pubchem.ncbi.nlm.nih.gov | |
Description | Data deposited in or computed by PubChem | |
CAS No. |
61093-23-0 | |
Record name | c-Di-GMP | |
Source | CAS Common Chemistry | |
URL | https://commonchemistry.cas.org/detail?cas_rn=61093-23-0 | |
Description | CAS Common Chemistry is an open community resource for accessing chemical information. Nearly 500,000 chemical substances from CAS REGISTRY cover areas of community interest, including common and frequently regulated chemicals, and those relevant to high school and undergraduate chemistry classes. This chemical information, curated by our expert scientists, is provided in alignment with our mission as a division of the American Chemical Society. | |
Explanation | The data from CAS Common Chemistry is provided under a CC-BY-NC 4.0 license, unless otherwise stated. | |
Retrosynthesis Analysis
AI-Powered Synthesis Planning: Our tool employs the Template_relevance Pistachio, Template_relevance Bkms_metabolic, Template_relevance Pistachio_ringbreaker, Template_relevance Reaxys, Template_relevance Reaxys_biocatalysis model, leveraging a vast database of chemical reactions to predict feasible synthetic routes.
One-Step Synthesis Focus: Specifically designed for one-step synthesis, it provides concise and direct routes for your target compounds, streamlining the synthesis process.
Accurate Predictions: Utilizing the extensive PISTACHIO, BKMS_METABOLIC, PISTACHIO_RINGBREAKER, REAXYS, REAXYS_BIOCATALYSIS database, our tool offers high-accuracy predictions, reflecting the latest in chemical research and data.
Strategy Settings
Precursor scoring | Relevance Heuristic |
---|---|
Min. plausibility | 0.01 |
Model | Template_relevance |
Template Set | Pistachio/Bkms_metabolic/Pistachio_ringbreaker/Reaxys/Reaxys_biocatalysis |
Top-N result to add to graph | 6 |
Feasible Synthetic Routes
Q1: What is cyclic diguanylic acid (c-di-GMP) and what is its significance in bacteria?
A: Cyclic diguanylic acid (this compound) is a ubiquitous second messenger molecule found in bacteria. [] It plays a crucial role in regulating a wide range of cellular processes in bacteria, primarily acting as a molecular switch between motile (free-swimming) and sessile (surface-attached) lifestyles. []
Q2: How is this compound synthesized and degraded within bacterial cells?
A: this compound is synthesized from two molecules of GTP by enzymes called diguanylate cyclases (DGCs), which contain a characteristic GGDEF domain. [, ] Conversely, this compound is degraded into linear pGpG or two GMP molecules by enzymes called phosphodiesterases (PDEs), which possess either EAL or HD-GYP domains. [, ]
Q3: How does the number of genes encoding this compound-modulating enzymes affect bacterial adaptation?
A: Bacteria exhibit significant variation in the number of genes encoding GGDEF, EAL, and HD-GYP proteins. [] A greater number of these genes translate into a more complex this compound signaling network, enabling bacteria to fine-tune the regulation of target genes and optimize their adaptation to diverse environments. [, ]
Q4: What is the general mechanism of action of this compound in bacterial signaling pathways?
A: this compound exerts its regulatory effects by binding to various effector proteins, such as transcription factors, enzymes, and multimeric protein complexes. [] This binding event triggers downstream signaling cascades that ultimately control a wide range of bacterial behaviors, including biofilm formation, motility, virulence, and cell cycle progression.
Q5: How does this compound influence biofilm formation?
A: High intracellular levels of this compound generally promote biofilm formation. [, , , ] This is achieved through multiple mechanisms, including:
- Repression of motility: this compound inhibits bacterial motility by reducing flagellar synthesis and function, thereby promoting surface attachment. [, , , ] For instance, in Pseudomonas aeruginosa, elevated this compound levels lead to reduced flagellar motility and enhanced biofilm formation. []
- Induction of biofilm matrix production: this compound stimulates the production of extracellular polysaccharide (EPS), a major component of the biofilm matrix. [, , ] For example, in Pseudomonas aeruginosa, the diguanylate cyclase RoeA promotes EPS production and contributes to biofilm formation. []
- Regulation of adhesin expression: this compound can modulate the expression of bacterial adhesins, which mediate attachment to surfaces and other cells. [] In Pseudomonas putida, this compound/FleQ regulates the transcription of lapE, which encodes an outer membrane pore protein involved in adhesin secretion and biofilm formation. []
Q6: How does this compound impact bacterial motility?
A: Elevated this compound levels typically suppress bacterial motility, primarily by inhibiting flagellar synthesis and function. [, , , ] For example, in Myxococcus xanthus, this compound regulates type IV pilus-dependent motility. [] Conversely, low this compound levels generally promote motility by facilitating flagellar biosynthesis and rotation. []
Q7: Does this compound regulate virulence in pathogenic bacteria?
A: The role of this compound in bacterial virulence is complex and can be context-dependent. While generally considered an inhibitor of virulence factors, exceptions to this paradigm exist. []
- Virulence suppression: In some pathogens, high this compound levels correlate with reduced virulence, often indirectly through the inhibition of motility and biofilm dispersal. [, , ] In Vibrio cholerae, for example, this compound directly inhibits the expression of cholera toxin. []
- Virulence enhancement: Conversely, in certain pathogens, this compound contributes to virulence by promoting host colonization, persistence, and immune evasion. [, , ] For instance, in Ehrlichia chaffeensis, this compound signaling is essential for bacterial invasion of human monocytes. []
Q8: Can you provide examples of specific this compound effector proteins and their functions?
A8: Numerous this compound effector proteins have been identified, each contributing to specific downstream effects:
- FleQ: In Pseudomonas species, FleQ acts as a transcriptional regulator, controlling the expression of biofilm- and flagellum-related genes in response to this compound. []
- BldD: In Streptomyces, BldD dimerizes upon binding to this compound, impacting the transcription of genes involved in differentiation and development. []
- PilZ domain proteins: These proteins, often containing one or more PilZ domains, are common this compound receptors involved in various cellular processes. [, , ] For instance, in Myxococcus xanthus, PlpA, PixA, and PixB are PilZ domain proteins with distinct roles in regulating motility and development. []
- MshE: This ATPase, associated with type IV pilus, binds this compound via its N-terminal T2SSE_N domain, influencing pilus-dependent processes. []
Q9: How is this compound signaling specificity achieved in bacteria with complex this compound networks?
A9: Maintaining signaling specificity in bacteria with numerous DGCs and PDEs is crucial for proper cellular function. Several mechanisms contribute to this specificity:
- Spatiotemporal regulation: The localization and timing of this compound synthesis and degradation are tightly controlled. [, , , ] Specific DGCs and PDEs are often localized to distinct cellular compartments or expressed under specific conditions, ensuring that this compound signals are directed to the appropriate downstream effectors.
- Protein-protein interactions: Direct interactions between this compound-metabolizing enzymes and their cognate effectors contribute to signaling fidelity. [, , , ] These interactions can enhance the local concentration of this compound near its target, preventing cross-talk between different pathways.
- Feedback regulation: this compound can modulate the activity of DGCs and PDEs through feedback mechanisms, fine-tuning its own intracellular levels. [] This feedback control helps to maintain this compound homeostasis and prevent aberrant signaling.
Q10: How does the study of this compound signaling contribute to our understanding of bacterial pathogenesis and potential therapeutic interventions?
A10: Understanding the intricacies of this compound signaling in pathogenic bacteria holds significant promise for developing novel therapeutic strategies:
- Anti-biofilm agents: Disrupting this compound signaling pathways essential for biofilm formation could lead to new treatments for chronic and recurrent infections. [, ] Targeting DGCs, PDEs, or this compound effector proteins could potentially prevent biofilm formation or disperse existing biofilms, rendering bacteria more susceptible to antibiotics and host immune responses.
- Antivirulence therapies: Modulating this compound signaling in pathogens where it promotes virulence could attenuate their pathogenicity and enhance the efficacy of existing treatments. [] Inhibiting specific DGCs or activating specific PDEs involved in virulence regulation could potentially disarm pathogens without directly killing them, thereby minimizing the emergence of antibiotic resistance.
- Probiotic interventions: Manipulating this compound signaling in beneficial bacteria could enhance their ability to colonize the host and outcompete pathogens. [] Increasing this compound levels in probiotics could potentially enhance their biofilm-forming capacity, improving their persistence and effectiveness in the gut.
試験管内研究製品の免責事項と情報
BenchChemで提示されるすべての記事および製品情報は、情報提供を目的としています。BenchChemで購入可能な製品は、生体外研究のために特別に設計されています。生体外研究は、ラテン語の "in glass" に由来し、生物体の外で行われる実験を指します。これらの製品は医薬品または薬として分類されておらず、FDAから任何の医療状態、病気、または疾患の予防、治療、または治癒のために承認されていません。これらの製品を人間または動物に体内に導入する形態は、法律により厳格に禁止されています。これらのガイドラインに従うことは、研究と実験において法的および倫理的な基準の遵守を確実にするために重要です。