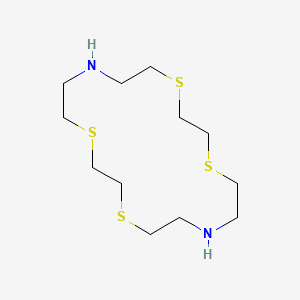
1,4,10,13-Tetrathia-7,16-diazacyclooctadecane
概要
説明
1,4,10,13-Tetrathia-7,16-diazacyclooctadecane (C₁₂H₂₆N₂S₄) is an 18-membered macrocyclic compound containing four sulfur (thia) and two nitrogen (aza) atoms within its heterocyclic ring. Its IUPAC name reflects the alternating thia and aza groups, with sulfur atoms at positions 1, 4, 10, and 13, and nitrogen atoms at positions 7 and 16 . The compound is also known by alternative names such as "Kryptofix 22" or "Cryptand 22" due to its cryptand-like structure .
準備方法
Synthetic Routes for 1,4,10,13-Tetrathia-7,16-diazacyclooctadecane
General Strategy
The synthesis typically proceeds via the formation of a macrocyclic dione intermediate, followed by reduction to the target macrocycle. The key steps include:
Macrocyclization : Formation of the macrocyclic ring by reacting dithiol and diamine precursors under high dilution conditions to favor intramolecular cyclization over polymerization.
Reduction : Conversion of the macrocyclic dione to the corresponding diamine macrocycle by borane reduction.
This approach leverages the nucleophilicity of sulfur and nitrogen atoms and the flexibility of the alkyl chains to achieve ring closure.
Representative Preparation Procedure
A detailed example from recent literature illustrates the preparation as follows:
-
- React 1,2-ethanedithiol (1.05 equivalents) with a diamine precursor (1 equivalent) in the presence of potassium carbonate (4 equivalents) in acetonitrile solvent.
- The mixture is refluxed under argon for 48 hours to promote macrocyclization.
- After reaction completion (monitored by mass spectrometry), the solvent is removed, and the crude product is washed with water to remove excess base and impurities.
- This yields this compound-6,17-dione as a solid intermediate.
Reduction to Macrocyclic Diamine
- The macrocyclic dione is suspended in dry tetrahydrofuran (THF), and an excess of borane-tetrahydrofuran complex (approximately 5.4 equivalents) is added under argon.
- The reaction mixture is refluxed overnight to reduce the dione to the diamine.
- After cooling, the reaction is quenched slowly with water, and volatiles are removed under vacuum.
- The residue is treated with 6 M hydrochloric acid and refluxed to ensure complete conversion and purification.
- The product is isolated as this compound with high purity.
This procedure is adapted and optimized from the synthesis reported in Dalton Transactions (2024).
Reaction Conditions and Parameters
Step | Reagents/Conditions | Time | Temperature | Notes |
---|---|---|---|---|
Macrocyclization | 1,2-ethanedithiol, K2CO3, acetonitrile, argon | 48 hours | Reflux (~82 °C) | High dilution favors cyclization |
Reduction | Borane-THF complex (5.4 eq.), dry THF, argon | Overnight | Reflux (~66 °C) | Excess borane ensures complete reduction |
Acid treatment | 6 M HCl, reflux | 3 hours | Reflux (~100 °C) | Purification and removal of borane residues |
Alternative Preparation Approaches and Modifications
Use of Different Solvents : Acetonitrile is preferred for macrocyclization due to its polarity and ability to dissolve both organic and inorganic reagents, but other polar aprotic solvents like dimethylformamide (DMF) may also be used.
Base Selection : Potassium carbonate is standard for neutralizing acidic byproducts and promoting deprotonation of thiols; alternatives like sodium carbonate or organic bases have been tested with varying efficiencies.
Macrocyclization Techniques : High dilution techniques or slow addition methods are employed to minimize polymerization and favor intramolecular ring closure.
Purification : Recrystallization from appropriate solvents or chromatographic methods are used to isolate the pure macrocycle.
Research Findings and Characterization Data
NMR Spectroscopy : $$^{1}H$$ and $$^{13}C$$ NMR confirm the macrocycle structure, showing characteristic chemical shifts for methylene protons adjacent to sulfur and nitrogen atoms.
Mass Spectrometry : Confirms molecular weight and purity of intermediates and final products.
X-ray Crystallography : Provides definitive structural confirmation of the macrocyclic ring and donor atom arrangement.
Yield and Purity : Typical overall yields range from 50% to 70% for the two-step process, with purity >95% after purification.
Summary Table of Preparation Methods
化学反応の分析
Types of Reactions
1,4,10,13-Tetrathia-7,16-diazacyclooctadecane undergoes various types of chemical reactions, including:
Oxidation: The sulfur atoms in the compound can be oxidized to form sulfoxides or sulfones.
Reduction: The compound can be reduced to form thiols or other reduced sulfur species.
Substitution: The nitrogen atoms can participate in nucleophilic substitution reactions.
Common Reagents and Conditions
Oxidation: Common oxidizing agents include hydrogen peroxide and m-chloroperbenzoic acid.
Reduction: Reducing agents such as lithium aluminum hydride or sodium borohydride are often used.
Substitution: Nucleophiles like amines or thiols can be used in substitution reactions.
Major Products Formed
Oxidation: Sulfoxides and sulfones.
Reduction: Thiols and other reduced sulfur species.
Substitution: Various substituted derivatives depending on the nucleophile used.
科学的研究の応用
Coordination Chemistry
Ligand Properties
TDA acts as a chelating ligand capable of forming stable complexes with various metal ions. Its structure allows for the coordination of metal ions through the sulfur and nitrogen atoms, facilitating the formation of diverse metal-ligand complexes. For example, studies have shown that TDA can form complexes with palladium(II), which are useful in catalysis and materials science .
Case Study: Metal Complex Formation
A significant study involved the synthesis of palladium complexes with TDA, where the ligand demonstrated effective coordination leading to the formation of extended polyiodide arrays. The structural analysis revealed that TDA's coordination environment significantly influences the properties of the resulting complexes .
Biological Applications
Metalloprotein Studies
TDA's ability to form stable metal complexes makes it a valuable tool in biological research, particularly in studying metalloproteins. These proteins often require metal ions for their biological activity, and TDA can mimic the natural binding sites found in these proteins .
Drug Delivery Systems
Research is ongoing into TDA's potential in drug delivery systems. Its ability to form stable complexes with therapeutic agents can enhance the solubility and bioavailability of drugs. Preliminary studies suggest that TDA could be utilized to transport metal-based drugs effectively .
Medical Applications
Radiopharmaceuticals
TDA has been investigated for use in radiopharmaceuticals due to its chelation properties. It can bind radioactive isotopes, making it suitable for cancer diagnosis and treatment. The stability of TDA-metal complexes is critical for ensuring that radioactive isotopes remain bound during biological processes .
Case Study: Strontium Mobilization
In a study aimed at addressing radioactive strontium incorporation in biological systems, derivatives of TDA were synthesized and evaluated for their ability to mobilize strontium ions from biological tissues. The results indicated that TDA derivatives could effectively chelate strontium, suggesting potential applications in radiation therapy .
Industrial Applications
Material Development
TDA is being explored for its role in developing new materials with enhanced properties such as conductivity and catalytic activity. Its unique sulfur-rich structure allows for modifications that can lead to materials with specific functionalities suitable for industrial applications .
作用機序
The mechanism by which 1,4,10,13-Tetrathia-7,16-diazacyclooctadecane exerts its effects is primarily through its ability to form stable complexes with metal ions. The nitrogen and sulfur atoms in the ring structure act as donor atoms, coordinating with metal ions to form stable chelates. These chelates can then participate in various chemical reactions, depending on the nature of the metal ion and the reaction conditions .
類似化合物との比較
Comparison with Similar Macrocyclic Compounds
Structural Comparisons
Table 1: Structural and Functional Differences
Metal Selectivity and Binding Mechanisms
This compound :
- Primarily used for lanthanide extraction via synergistic systems. The sulfur atoms enhance electron-donating capacity, while lauric acid acts as a synergist, improving extraction efficiency for hard Lewis acids like La³⁺ and Nd³⁺ .
- Despite sulfur's typical affinity for soft metals (e.g., Hg²⁺), the synergistic system shifts selectivity toward lanthanides .
N,N'-Dibenzyl-tetraoxa-diazacyclooctadecane :
18-Crown-6 :
DTODC :
Conformational Flexibility and Stability
This compound :
N,N'-Dibenzyl-tetraoxa-diazacyclooctadecane :
Dithiophenediazacrown ethers :
Research Findings and Performance Metrics
Table 2: Comparative Performance in Metal Extraction/Sensing
生物活性
1,4,10,13-Tetrathia-7,16-diazacyclooctadecane (often abbreviated as aneN2S4 ) is a macrocyclic compound notable for its unique structural characteristics and biological activities. This compound has garnered interest due to its potential applications in medicinal chemistry, particularly in the development of metal complexes that exhibit significant biological activity.
Structural Characteristics
The compound features a macrocyclic structure comprising four sulfur atoms and two nitrogen atoms within an 18-membered ring. This configuration allows for the formation of stable complexes with various metal ions, which can influence its biological properties.
Metal Ion Complexation
This compound has been shown to effectively form complexes with various metal ions. The biological activity of these complexes often depends on the type of metal ion involved. For example:
- Palladium Complexes : The complexation of palladium with aneN2S4 has been studied extensively. Research indicates that palladium(II) complexes can exhibit antitumor activity by inducing apoptosis in cancer cells .
- Europium Complexes : Studies have demonstrated that europium complexes derived from aneN2S4 show luminescent properties that can be utilized in bioimaging applications .
Antioxidant Properties
The antioxidant activity of this compound and its metal complexes has been evaluated through various assays. These compounds have shown the ability to scavenge free radicals effectively, which is crucial in preventing oxidative stress-related diseases .
Case Study 1: Antitumor Activity
A study investigated the antitumor effects of palladium(II) complexes formed with this compound. The results indicated a significant reduction in cell viability in human cancer cell lines when treated with these complexes. The mechanism was linked to the induction of apoptosis and cell cycle arrest .
Case Study 2: Antioxidant Activity
Another research focused on the antioxidant potential of aneN2S4 and its complexes. The study utilized DPPH (2,2-diphenyl-1-picrylhydrazyl) and ABTS (2,2'-azino-bis(3-ethylbenzothiazoline-6-sulfonic acid)) assays to evaluate the scavenging ability of these compounds. The findings revealed that the presence of metal ions significantly enhanced the antioxidant activity compared to the free ligand .
Table 1: Summary of Biological Activities
Q & A
Basic Research Questions
Q. What are the key spectroscopic techniques for characterizing 1,4,10,13-Tetrathia-7,16-diazacyclooctadecane, and how are they applied?
- Methodological Answer :
- Nuclear Magnetic Resonance (NMR) : 1H and 13C NMR are critical for confirming the macrocyclic structure. For example, proton signals for thioether (-S-) and amine (-NH-) groups appear in distinct regions (δ 2.5–3.5 ppm and δ 1.5–2.5 ppm, respectively). Integration ratios verify stoichiometry .
- X-ray Crystallography : Single-crystal diffraction resolves spatial conformation, bond angles, and coordination geometry. Evidence from analogous compounds (e.g., 1,4,10,13-Tetraoxa-7,16-diazacyclooctadecane) shows crown ether-like structures with nitrogen and sulfur atoms in donor positions .
- Mass Spectrometry (MS) : High-resolution MS (HRMS) confirms molecular weight and fragmentation patterns, particularly for sulfur-rich macrocycles prone to oxidation .
Q. How is this compound synthesized, and what are common pitfalls?
- Methodological Answer :
- Cyclocondensation : React dithiols with diamines under templated conditions (e.g., metal ions like Zn²⁺ or Cu²⁺). Slow addition of reactants in anhydrous THF at 60–80°C minimizes disulfide byproducts.
- Purification Challenges : Column chromatography (silica gel, eluent: CH₂Cl₂/MeOH) is essential due to polar byproducts. Analogous tetraoxa macrocycles require rigorous drying to prevent hydrolysis .
Q. What experimental strategies ensure stability during ligand-complexation studies?
- Methodological Answer :
- pH Control : Use buffered solutions (e.g., phosphate buffer, pH 7.0–7.4) to prevent protonation of amine donors.
- Oxygen Exclusion : Conduct reactions under nitrogen/argon to avoid sulfur oxidation. Electrochemical studies of similar ligands show redox activity at -0.2 V to +0.5 V (vs. Ag/AgCl) .
Q. How do researchers design ligand derivatives for selective metal binding?
- Methodological Answer :
- Functionalization : Introduce electron-withdrawing groups (e.g., nitro or carbonyl) to sulfur or nitrogen donors to enhance affinity for soft metals (e.g., Pd²⁺, Pt²⁺). For example, 7,16-bis(5-t-butyl-2-hydroxybenzyl) derivatives improve Zr⁴⁺ binding in hydrolysis studies .
- Competition Experiments : Titrate metal mixtures (e.g., Cu²⁺/Zn²⁺) and monitor selectivity via UV-Vis or fluorescence quenching .
Q. What are effective literature-search strategies for this compound?
- Methodological Answer :
- Automated Tools : Use APIs (e.g., Google Scholar, PubMed) with keywords: "tetrathia diazacyclooctadecane," "sulfur macrocycles," "coordination chemistry."
- Gray Literature : Search patents and government reports (e.g., EPA databases) for toxicity or synthesis protocols. Prioritize peer-reviewed journals over commercial databases .
Advanced Research Questions
Q. How can computational chemistry optimize reaction pathways for synthesizing this macrocycle?
- Methodological Answer :
- Quantum Mechanical Calculations : Density Functional Theory (DFT) models transition states for cyclization. For example, B3LYP/6-31G* level calculations predict energy barriers for dithiol-amine condensation .
- Molecular Dynamics (MD) : Simulate solvent effects (e.g., THF vs. DMF) on templating efficiency. Adjust force fields to account for sulfur’s polarizability .
Q. What methodologies resolve contradictions in reported coordination geometries?
- Methodological Answer :
- Variable-Temperature NMR : Detect dynamic equilibria (e.g., chair-boat conformational changes) in solution.
- Extended X-ray Absorption Fine Structure (EXAFS) : Resolve metal-ligand bond lengths in amorphous or poorly crystalline samples. Compare with crystallographic data from analogous compounds .
Q. How do researchers analyze conflicting data in sulfur oxidation studies?
- Methodological Answer :
- Controlled Replication : Repeat experiments under strict inert atmospheres (glovebox) to isolate oxidation pathways.
- Electron Paramagnetic Resonance (EPR) : Identify radical intermediates during oxidation. For thioethers, g-values ≈ 2.03 indicate sulfur-centered radicals .
Q. What advanced techniques compare the macrocycle’s reactivity with oxygen/sulfur analogs?
- Methodological Answer :
- Kinetic Isotope Effects (KIE) : Replace sulfur with 34S to study bond cleavage rates in hydrolysis. Compare with tetraoxa analogs (e.g., 1,10-Diaza-18-crown-6) to quantify electronic effects .
- Cyclic Voltammetry (CV) : Measure redox potentials of sulfur vs. oxygen donors. Thia crowns typically show lower oxidation potentials due to sulfur’s lower electronegativity .
Q. How can factorial design improve catalytic studies involving this macrocycle?
- Methodological Answer :
- Full Factorial Design : Vary factors like pH, temperature, and metal concentration (e.g., 2³ design) to identify interactions. Use ANOVA to rank significance.
- Response Surface Methodology (RSM) : Optimize conditions for maximum catalytic turnover (e.g., Zr⁴⁺-mediated peptide hydrolysis). Central Composite Design (CCD) reduces experimental runs by 40% .
特性
IUPAC Name |
1,4,10,13-tetrathia-7,16-diazacyclooctadecane | |
---|---|---|
Source | PubChem | |
URL | https://pubchem.ncbi.nlm.nih.gov | |
Description | Data deposited in or computed by PubChem | |
InChI |
InChI=1S/C12H26N2S4/c1-5-15-9-10-17-7-3-14-4-8-18-12-11-16-6-2-13-1/h13-14H,1-12H2 | |
Source | PubChem | |
URL | https://pubchem.ncbi.nlm.nih.gov | |
Description | Data deposited in or computed by PubChem | |
InChI Key |
HDQRZGDWLMSBSD-UHFFFAOYSA-N | |
Source | PubChem | |
URL | https://pubchem.ncbi.nlm.nih.gov | |
Description | Data deposited in or computed by PubChem | |
Canonical SMILES |
C1CSCCSCCNCCSCCSCCN1 | |
Source | PubChem | |
URL | https://pubchem.ncbi.nlm.nih.gov | |
Description | Data deposited in or computed by PubChem | |
Molecular Formula |
C12H26N2S4 | |
Source | PubChem | |
URL | https://pubchem.ncbi.nlm.nih.gov | |
Description | Data deposited in or computed by PubChem | |
DSSTOX Substance ID |
DTXSID10339974 | |
Record name | 1,4,10,13-Tetrathia-7,16-diazacyclooctadecane | |
Source | EPA DSSTox | |
URL | https://comptox.epa.gov/dashboard/DTXSID10339974 | |
Description | DSSTox provides a high quality public chemistry resource for supporting improved predictive toxicology. | |
Molecular Weight |
326.6 g/mol | |
Source | PubChem | |
URL | https://pubchem.ncbi.nlm.nih.gov | |
Description | Data deposited in or computed by PubChem | |
CAS No. |
20934-69-4 | |
Record name | 1,4,10,13-Tetrathia-7,16-diazacyclooctadecane | |
Source | EPA DSSTox | |
URL | https://comptox.epa.gov/dashboard/DTXSID10339974 | |
Description | DSSTox provides a high quality public chemistry resource for supporting improved predictive toxicology. | |
Synthesis routes and methods
Procedure details
Retrosynthesis Analysis
AI-Powered Synthesis Planning: Our tool employs the Template_relevance Pistachio, Template_relevance Bkms_metabolic, Template_relevance Pistachio_ringbreaker, Template_relevance Reaxys, Template_relevance Reaxys_biocatalysis model, leveraging a vast database of chemical reactions to predict feasible synthetic routes.
One-Step Synthesis Focus: Specifically designed for one-step synthesis, it provides concise and direct routes for your target compounds, streamlining the synthesis process.
Accurate Predictions: Utilizing the extensive PISTACHIO, BKMS_METABOLIC, PISTACHIO_RINGBREAKER, REAXYS, REAXYS_BIOCATALYSIS database, our tool offers high-accuracy predictions, reflecting the latest in chemical research and data.
Strategy Settings
Precursor scoring | Relevance Heuristic |
---|---|
Min. plausibility | 0.01 |
Model | Template_relevance |
Template Set | Pistachio/Bkms_metabolic/Pistachio_ringbreaker/Reaxys/Reaxys_biocatalysis |
Top-N result to add to graph | 6 |
Feasible Synthetic Routes
試験管内研究製品の免責事項と情報
BenchChemで提示されるすべての記事および製品情報は、情報提供を目的としています。BenchChemで購入可能な製品は、生体外研究のために特別に設計されています。生体外研究は、ラテン語の "in glass" に由来し、生物体の外で行われる実験を指します。これらの製品は医薬品または薬として分類されておらず、FDAから任何の医療状態、病気、または疾患の予防、治療、または治癒のために承認されていません。これらの製品を人間または動物に体内に導入する形態は、法律により厳格に禁止されています。これらのガイドラインに従うことは、研究と実験において法的および倫理的な基準の遵守を確実にするために重要です。