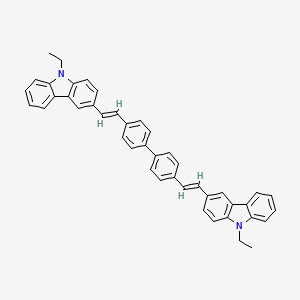
BCzVBi
概要
説明
4,4’-Bis(2-(9-ethyl-9H-carbazol-3-yl)vinyl)-1,1’-biphenyl is an organic compound that belongs to the class of carbazole derivatives. Carbazole derivatives are known for their unique electronic properties, making them valuable in various scientific and industrial applications. This compound, in particular, is of interest due to its potential use in organic electronics and optoelectronic devices.
科学的研究の応用
4,4’-Bis(2-(9-ethyl-9H-carbazol-3-yl)vinyl)-1,1’-biphenyl has several scientific research applications, including:
Organic Electronics: The compound is used in the development of organic light-emitting diodes (OLEDs) due to its excellent electron-transporting properties.
Photovoltaics: It is employed in organic photovoltaic cells as a donor material, contributing to the efficiency of light absorption and charge transport.
Biological Applications: Carbazole derivatives have shown potential in biological applications, such as anticancer agents and fluorescent probes for bioimaging.
Material Science: The compound is used in the synthesis of advanced materials with unique optical and electronic properties, making it valuable in the field of material science.
作用機序
Target of Action
The primary target of 4,4’-Bis(9-ethyl-3-carbazovinylene)-1,1’-biphenyl (BCzVBi) is the organic light-emitting diodes (OLEDs). This compound is used as a fluorescent emitter in OLEDs, mixed with two charge transport hosts . The efficiency of the device increases as the concentration of this compound increases .
Mode of Action
This compound interacts with its targets, the OLEDs, by being mixed with two charge transport hosts. The energy gap for electrons transporting from TPBi to this compound is higher than the energy gap from TPBi to CBP, showing that the electron injection efficiency from the ETL to the EML would be suppressed while the concentration of this compound increased for the device .
Biochemical Pathways
The balance of the carriers and the distribution of the exciton could be improved via this D-EML structure in two situations, which could improve the performance of the device .
Pharmacokinetics
It is known that the device efficiency increases as this compound concentration increases , suggesting that the concentration of this compound in the device may influence its bioavailability.
Result of Action
The molecular and cellular effects of this compound’s action result in an increase in the efficiency of the OLEDs. The luminance and luminous efficiency of BOLED doped this compound in EML and HTL/ETL improved significantly . This suggests that this compound has a significant impact on the performance of OLEDs.
準備方法
Synthetic Routes and Reaction Conditions
The synthesis of 4,4’-Bis(2-(9-ethyl-9H-carbazol-3-yl)vinyl)-1,1’-biphenyl typically involves a multi-step process. One common method is the Suzuki-Miyaura cross-coupling reaction. This reaction involves the coupling of a boronic acid derivative of 9-ethyl-9H-carbazole with a halogenated biphenyl compound. The reaction is usually carried out in the presence of a palladium catalyst and a base, such as potassium carbonate, in an organic solvent like toluene or dimethylformamide. The reaction conditions often include heating the mixture to a temperature of around 80-100°C for several hours to ensure complete conversion.
Industrial Production Methods
In an industrial setting, the production of 4,4’-Bis(2-(9-ethyl-9H-carbazol-3-yl)vinyl)-1,1’-biphenyl may involve scaling up the Suzuki-Miyaura cross-coupling reaction. This requires optimizing the reaction conditions to achieve high yields and purity. Continuous flow reactors and automated synthesis platforms can be employed to enhance the efficiency and reproducibility of the process. Additionally, purification techniques such as column chromatography and recrystallization are used to isolate the final product.
化学反応の分析
Types of Reactions
4,4’-Bis(2-(9-ethyl-9H-carbazol-3-yl)vinyl)-1,1’-biphenyl can undergo various chemical reactions, including:
Oxidation: The compound can be oxidized using oxidizing agents like potassium permanganate or chromium trioxide, leading to the formation of corresponding carbazole-quinone derivatives.
Reduction: Reduction reactions can be carried out using reducing agents such as sodium borohydride or lithium aluminum hydride, resulting in the formation of reduced carbazole derivatives.
Substitution: Electrophilic substitution reactions can occur at the carbazole moiety, allowing for the introduction of various functional groups. Common reagents for these reactions include halogens, nitrating agents, and sulfonating agents.
Common Reagents and Conditions
Oxidation: Potassium permanganate in an acidic medium, chromium trioxide in acetic acid.
Reduction: Sodium borohydride in methanol, lithium aluminum hydride in tetrahydrofuran.
Substitution: Halogens (chlorine, bromine) in the presence of a Lewis acid catalyst, nitrating agents (nitric acid) in sulfuric acid, sulfonating agents (sulfur trioxide) in fuming sulfuric acid.
Major Products Formed
Oxidation: Carbazole-quinone derivatives.
Reduction: Reduced carbazole derivatives.
Substitution: Halogenated, nitrated, or sulfonated carbazole derivatives.
類似化合物との比較
Similar Compounds
- 4,4’-Bis(2-(9-hexyl-9H-carbazol-3-yl)vinyl)-1,1’-biphenyl
- 4,4’-Bis(2-(9-phenyl-9H-carbazol-3-yl)vinyl)-1,1’-biphenyl
- 4,4’-Bis(2-(9-methyl-9H-carbazol-3-yl)vinyl)-1,1’-biphenyl
Uniqueness
4,4’-Bis(2-(9-ethyl-9H-carbazol-3-yl)vinyl)-1,1’-biphenyl is unique due to its specific electronic properties conferred by the ethyl group on the carbazole moiety. This modification enhances its solubility and processability, making it more suitable for certain applications compared to its analogs. Additionally, the compound’s ability to form stable π-π interactions and its high thermal stability contribute to its distinct advantages in optoelectronic devices.
特性
IUPAC Name |
9-ethyl-3-[(E)-2-[4-[4-[(E)-2-(9-ethylcarbazol-3-yl)ethenyl]phenyl]phenyl]ethenyl]carbazole | |
---|---|---|
Source | PubChem | |
URL | https://pubchem.ncbi.nlm.nih.gov | |
Description | Data deposited in or computed by PubChem | |
InChI |
InChI=1S/C44H36N2/c1-3-45-41-11-7-5-9-37(41)39-29-33(21-27-43(39)45)15-13-31-17-23-35(24-18-31)36-25-19-32(20-26-36)14-16-34-22-28-44-40(30-34)38-10-6-8-12-42(38)46(44)4-2/h5-30H,3-4H2,1-2H3/b15-13+,16-14+ | |
Source | PubChem | |
URL | https://pubchem.ncbi.nlm.nih.gov | |
Description | Data deposited in or computed by PubChem | |
InChI Key |
RAPHUPWIHDYTKU-WXUKJITCSA-N | |
Source | PubChem | |
URL | https://pubchem.ncbi.nlm.nih.gov | |
Description | Data deposited in or computed by PubChem | |
Canonical SMILES |
CCN1C2=C(C=C(C=C2)C=CC3=CC=C(C=C3)C4=CC=C(C=C4)C=CC5=CC6=C(C=C5)N(C7=CC=CC=C76)CC)C8=CC=CC=C81 | |
Source | PubChem | |
URL | https://pubchem.ncbi.nlm.nih.gov | |
Description | Data deposited in or computed by PubChem | |
Isomeric SMILES |
CCN1C2=CC=CC=C2C3=C1C=CC(=C3)/C=C/C4=CC=C(C=C4)C5=CC=C(C=C5)/C=C/C6=CC7=C(N(C8=CC=CC=C78)CC)C=C6 | |
Source | PubChem | |
URL | https://pubchem.ncbi.nlm.nih.gov | |
Description | Data deposited in or computed by PubChem | |
Molecular Formula |
C44H36N2 | |
Source | PubChem | |
URL | https://pubchem.ncbi.nlm.nih.gov | |
Description | Data deposited in or computed by PubChem | |
DSSTOX Substance ID |
DTXSID10626230 | |
Record name | 3,3'-{[1,1'-Biphenyl]-4,4'-diyldi[(E)ethene-2,1-diyl]}bis(9-ethyl-9H-carbazole) | |
Source | EPA DSSTox | |
URL | https://comptox.epa.gov/dashboard/DTXSID10626230 | |
Description | DSSTox provides a high quality public chemistry resource for supporting improved predictive toxicology. | |
Molecular Weight |
592.8 g/mol | |
Source | PubChem | |
URL | https://pubchem.ncbi.nlm.nih.gov | |
Description | Data deposited in or computed by PubChem | |
CAS No. |
475480-90-1 | |
Record name | 3,3'-{[1,1'-Biphenyl]-4,4'-diyldi[(E)ethene-2,1-diyl]}bis(9-ethyl-9H-carbazole) | |
Source | EPA DSSTox | |
URL | https://comptox.epa.gov/dashboard/DTXSID10626230 | |
Description | DSSTox provides a high quality public chemistry resource for supporting improved predictive toxicology. | |
Retrosynthesis Analysis
AI-Powered Synthesis Planning: Our tool employs the Template_relevance Pistachio, Template_relevance Bkms_metabolic, Template_relevance Pistachio_ringbreaker, Template_relevance Reaxys, Template_relevance Reaxys_biocatalysis model, leveraging a vast database of chemical reactions to predict feasible synthetic routes.
One-Step Synthesis Focus: Specifically designed for one-step synthesis, it provides concise and direct routes for your target compounds, streamlining the synthesis process.
Accurate Predictions: Utilizing the extensive PISTACHIO, BKMS_METABOLIC, PISTACHIO_RINGBREAKER, REAXYS, REAXYS_BIOCATALYSIS database, our tool offers high-accuracy predictions, reflecting the latest in chemical research and data.
Strategy Settings
Precursor scoring | Relevance Heuristic |
---|---|
Min. plausibility | 0.01 |
Model | Template_relevance |
Template Set | Pistachio/Bkms_metabolic/Pistachio_ringbreaker/Reaxys/Reaxys_biocatalysis |
Top-N result to add to graph | 6 |
Feasible Synthetic Routes
Q1: What is the molecular formula and weight of BCzVBi?
A1: The molecular formula of this compound is C40H30N2, and its molecular weight is 546.69 g/mol.
Q2: What spectroscopic data is available for this compound?
A2: Several studies provide spectroscopic data for this compound. Information on its UV-Vis absorption and photoluminescence (PL) spectra is readily available. For instance, researchers observed that the PL spectrum of DNBN shows a better overlap with the UV-Vis absorption spectrum of this compound compared to the PL spectrum of MADN. [] This overlap suggests efficient energy transfer from DNBN to this compound.
Q3: What is the energy bandgap of this compound?
A3: The energy bandgap of this compound is reported to be 3.0 eV. []
Q4: How does the molecular structure of this compound contribute to its deep blue emission?
A4: The conjugated structure of this compound, featuring alternating single and double bonds, allows for electron delocalization across the molecule. This delocalization results in a wider energy gap between the highest occupied molecular orbital (HOMO) and the lowest unoccupied molecular orbital (LUMO), leading to blue light emission upon relaxation from the excited state.
Q5: What are the primary applications of this compound in OLEDs?
A5: this compound is primarily used as a deep-blue fluorescent dopant in the emissive layer of OLEDs. [, , , , ]
Q6: Which host materials are commonly used with this compound in OLEDs?
A6: Several host materials have been investigated for use with this compound, including:
- 4′-(dinaphthalen-2-yl)-1,1′-binaphthyl (DNBN) [, ]
- 2-methyl-9,10-di(2-naphthyl) anthracene (MADN) [, , , ]
- 1,4-(dinaphthalen-2-yl)-naphthalene (DNN) []
- 4,4-bis(2,2-diphenylyinyl)-1,10-biphenyl (DPVBi) [, , , , ]
- 1,3-bis(carbazol-9-yl)benzene (mCP) [, ]
Q7: How does the choice of host material impact the performance of this compound-based OLEDs?
A7: The choice of host material significantly influences the performance of this compound-based OLEDs. Factors like energy level alignment, energy transfer efficiency, and charge carrier mobility of the host are crucial. For instance, DNBN, with its favorable energy level alignment and efficient energy transfer to this compound, leads to enhanced device efficiency. []
Q8: What are the advantages of using this compound as a blue emitter compared to other materials?
A8: this compound offers several advantages as a blue emitter:
- Deep blue emission: It enables the fabrication of deep-blue OLEDs, crucial for achieving a wider color gamut in displays. [, , ]
- High efficiency: When paired with suitable host materials, this compound can lead to highly efficient OLEDs, contributing to lower power consumption. [, , , ]
- Good color stability: Research has shown that this compound-based devices can exhibit good color stability even at high luminance levels. [, ]
Q9: What are the challenges associated with using this compound in OLEDs?
A9: Despite its advantages, this compound also presents some challenges:
- Concentration quenching: At higher concentrations, this compound tends to aggregate, leading to a decrease in fluorescence efficiency. []
- Deep blue emission optimization: Achieving efficient and stable deep-blue emission with the desired CIE coordinates remains an ongoing challenge. [, ]
Q10: How does the device structure influence the performance of this compound-based OLEDs?
A10: The device structure significantly impacts OLED performance. Researchers have explored various strategies to optimize this compound-based devices:
- Doped transport layers: Incorporating this compound into the hole transport layer (HTL) and electron transport layer (ETL) has been shown to improve luminance and luminous efficiency by influencing charge carrier balance and exciton generation. [, ]
- Double emission layers (D-EMLs): Utilizing D-EMLs with this compound doped in both layers can broaden the recombination zone and improve charge carrier confinement, enhancing efficiency. []
- Quantum well structures: Employing a quantum well structure in the emissive layer has demonstrated improvements in luminance and luminous efficiency. []
Q11: What is the role of aluminum nanoparticles (Al-NPs) in enhancing the luminescence efficiency of this compound-based OLEDs?
A11: Inserting Al-NPs into the electron transport layer of this compound-based OLEDs can enhance luminescence efficiency through the local surface plasmon polariton (LSPP) effect. [] The LSPP effect, excited in Al-NPs by the emitted light from this compound, can enhance the radiative decay rate of the emitter, leading to increased efficiency.
Q12: How does the distance between Al-NPs and the this compound emitting layer impact OLED performance?
A12: The distance between the Al-NPs and the this compound emitting layer is crucial for optimal LSPP coupling and device performance. At very close proximity, fluorescence quenching can occur, while at larger distances, the LSPP effect weakens due to attenuated radiation from this compound. [] Finding the optimal distance is key to maximizing the LSPP-enhanced efficiency.
試験管内研究製品の免責事項と情報
BenchChemで提示されるすべての記事および製品情報は、情報提供を目的としています。BenchChemで購入可能な製品は、生体外研究のために特別に設計されています。生体外研究は、ラテン語の "in glass" に由来し、生物体の外で行われる実験を指します。これらの製品は医薬品または薬として分類されておらず、FDAから任何の医療状態、病気、または疾患の予防、治療、または治癒のために承認されていません。これらの製品を人間または動物に体内に導入する形態は、法律により厳格に禁止されています。これらのガイドラインに従うことは、研究と実験において法的および倫理的な基準の遵守を確実にするために重要です。