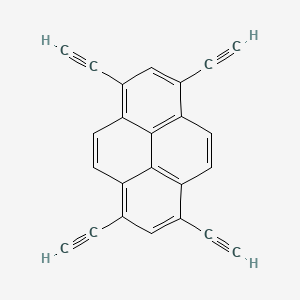
1,3,6,8-テトラエチニルピレン
概要
説明
1,3,6,8-Tetraethynylpyrene is a polycyclic aromatic hydrocarbon with four ethynyl groups attached to the pyrene core
科学的研究の応用
Photophysical Properties and Applications
TEP exhibits remarkable photophysical characteristics, making it suitable for applications in fluorescence and optoelectronics. The incorporation of TEP into polymer matrices enhances the luminescence properties of the resulting materials.
- Fluorescent Probes : TEP can be utilized as a fluorescent probe in biological imaging due to its high quantum yield and stability under UV light. Studies have shown that TEP derivatives with triazole units at specific positions exhibit enhanced fluorescence properties, making them ideal for bioimaging applications .
- Organic Light Emitting Diodes (OLEDs) : The high photoluminescence efficiency of TEP makes it a candidate for use in OLEDs. Its ability to emit bright blue light is particularly valuable in display technologies .
Energy Storage Materials
TEP has been integrated into the development of advanced energy storage materials, notably in supercapacitors and batteries.
- Supercapacitors : Research indicates that TEP-based porous organic polymers can achieve high capacitance values. For instance, a study reported a capacitance retention of 95% after 2000 charge-discharge cycles when TEP was incorporated into a composite material . This demonstrates TEP's potential for enhancing the performance of supercapacitors.
- CO2 Capture : TEP has also been utilized in the synthesis of microporous carbon materials designed for selective CO2 capture. The incorporation of TEP into these materials significantly improves their adsorption capacity and thermal stability .
Synthesis of Functional Materials
The versatility of TEP in chemical synthesis allows for the creation of functional materials with tailored properties.
- Polymer Composites : TEP is often used in the synthesis of polymer composites through reactions such as Sonogashira coupling. These composites can exhibit enhanced mechanical and thermal properties, making them suitable for various industrial applications .
- Nanostructured Materials : The use of TEP in creating nanostructured materials has been explored, particularly in the context of enhancing electronic properties. Such materials can be employed in sensors and other electronic devices due to their improved conductivity and surface area .
Case Study 1: Photophysical Characterization
A study focused on the photophysical behavior of triazole-substituted TEP derivatives revealed their potential as efficient fluorescent markers. The derivatives demonstrated significant shifts in emission spectra based on substituent variations, showcasing their tunable photophysical properties .
Case Study 2: Supercapacitor Development
In another research project, a microporous carbon material synthesized using TEP exhibited exceptional energy storage capabilities. The material showed a specific capacitance of 735 F/g and maintained performance over extended cycling tests, indicating its viability for commercial supercapacitor applications .
Data Summary Table
作用機序
Target of Action
1,3,6,8-Tetraethynylpyrene is a tetra-substituted derivative of pyrene . It is primarily targeted towards light absorption and emission due to its extended π conjugation through acetylenic linkages . This makes it a potential candidate for use in optoelectronic devices and as a fluorescent probe .
Mode of Action
The mode of action of 1,3,6,8-Tetraethynylpyrene is primarily through its interaction with light. It exhibits a strongly allowed S0–S1 transition, which is a type of electronic transition in molecules that involves the absorption or emission of light . This property is influenced by the solvent polarity, which mediates vibronic coupling and therefore the emission band intensities .
Biochemical Pathways
The biochemical pathways affected by 1,3,6,8-Tetraethynylpyrene are related to light absorption and emission. The compound’s extended acetylenic conjugation influences its absorption and fluorescence emission characteristics . This results in a significant redshift in the absorption and fluorescence emission bands .
Pharmacokinetics
Its solubility in common organic solvents like ethanol and dimethylformamide suggests it may have good bioavailability .
Result of Action
The result of the action of 1,3,6,8-Tetraethynylpyrene is the emission of fluorescence in the visible light region (400–550 nm) . This is due to the compound’s strong fluorescence quantum yield and shorter fluorescence lifetime compared to pyrene .
Action Environment
The action of 1,3,6,8-Tetraethynylpyrene is influenced by environmental factors such as solvent polarity and temperature . . This suggests that the compound’s action, efficacy, and stability are relatively insensitive to changes in these environmental conditions.
生化学分析
Biochemical Properties
1,3,6,8-Tetraethynylpyrene plays a crucial role in biochemical reactions due to its ability to interact with various biomolecules. It has been observed to interact with enzymes, proteins, and other biomolecules through π-π interactions and hydrogen bonding. For instance, 1,3,6,8-Tetraethynylpyrene can bind to enzymes involved in oxidative stress responses, thereby modulating their activity. Additionally, its interaction with proteins can lead to conformational changes that affect protein function and stability .
Cellular Effects
The effects of 1,3,6,8-Tetraethynylpyrene on cellular processes are profound. It has been shown to influence cell signaling pathways, gene expression, and cellular metabolism. In particular, 1,3,6,8-Tetraethynylpyrene can activate or inhibit specific signaling pathways, leading to altered cellular responses. For example, it can modulate the expression of genes involved in cell proliferation and apoptosis, thereby impacting cell survival and growth . Furthermore, its presence in cells can affect metabolic flux, leading to changes in the levels of key metabolites.
Molecular Mechanism
At the molecular level, 1,3,6,8-Tetraethynylpyrene exerts its effects through various mechanisms. It can bind to biomolecules such as DNA, RNA, and proteins, influencing their function. The binding interactions of 1,3,6,8-Tetraethynylpyrene with enzymes can result in either inhibition or activation, depending on the enzyme’s role in cellular processes. Additionally, 1,3,6,8-Tetraethynylpyrene can induce changes in gene expression by interacting with transcription factors and other regulatory proteins .
Temporal Effects in Laboratory Settings
In laboratory settings, the effects of 1,3,6,8-Tetraethynylpyrene can change over time. Studies have shown that this compound is relatively stable under standard laboratory conditions, with minimal degradation observed over extended periods. Its long-term effects on cellular function can vary depending on the experimental conditions. For instance, prolonged exposure to 1,3,6,8-Tetraethynylpyrene can lead to cumulative changes in cellular metabolism and gene expression .
Dosage Effects in Animal Models
The effects of 1,3,6,8-Tetraethynylpyrene in animal models are dose-dependent. At low doses, it may exhibit minimal toxicity and can be used to study its biochemical interactions without adverse effects. At higher doses, 1,3,6,8-Tetraethynylpyrene can induce toxic effects, including oxidative stress and cellular damage. These threshold effects highlight the importance of careful dosage control in experimental studies .
Metabolic Pathways
1,3,6,8-Tetraethynylpyrene is involved in various metabolic pathways, interacting with enzymes and cofactors that facilitate its metabolism. It can undergo oxidative metabolism, leading to the formation of reactive intermediates that can further interact with cellular components. These metabolic processes can influence the overall metabolic flux and alter the levels of key metabolites in cells .
Transport and Distribution
The transport and distribution of 1,3,6,8-Tetraethynylpyrene within cells and tissues are mediated by specific transporters and binding proteins. Once inside the cell, it can localize to various cellular compartments, including the cytoplasm and nucleus. The distribution of 1,3,6,8-Tetraethynylpyrene can affect its biochemical activity and interactions with cellular components .
Subcellular Localization
1,3,6,8-Tetraethynylpyrene exhibits distinct subcellular localization patterns, which can influence its activity and function. It can be targeted to specific cellular compartments through post-translational modifications and targeting signals. For example, 1,3,6,8-Tetraethynylpyrene may localize to the mitochondria, where it can impact mitochondrial function and energy metabolism .
準備方法
Synthetic Routes and Reaction Conditions
1,3,6,8-Tetraethynylpyrene can be synthesized through various methods, including the Sonogashira coupling reaction, Corey–Fuchs reaction, and oxidative coupling (Eglinton coupling). One common method involves the following steps:
Sonogashira Coupling: This reaction involves the coupling of 1,3,6,8-tetrabromopyrene with trimethylsilylacetylene in the presence of a palladium catalyst ([PdCl2(PPh3)2]), copper iodide (CuI), and triphenylphosphine (PPh3) in a solvent mixture of diisopropylamine and tetrahydrofuran (THF) under nitrogen atmosphere at 60°C for 12 hours.
Deprotection: The trimethylsilyl groups are then removed using a fluoride source such as tetrabutylammonium fluoride (TBAF) to yield 1,3,6,8-tetraethynylpyrene.
Industrial Production Methods
Industrial production of 1,3,6,8-tetraethynylpyrene typically involves large-scale Sonogashira coupling reactions followed by deprotection steps. The reaction conditions are optimized to ensure high yield and purity of the final product.
化学反応の分析
Types of Reactions
1,3,6,8-Tetraethynylpyrene undergoes various chemical reactions, including:
Oxidation: The ethynyl groups can be oxidized to form carbonyl compounds.
Reduction: The ethynyl groups can be reduced to form alkenes or alkanes.
Substitution: The ethynyl groups can participate in substitution reactions to form various derivatives.
Common Reagents and Conditions
Oxidation: Common oxidizing agents include potassium permanganate (KMnO4) and osmium tetroxide (OsO4).
Reduction: Common reducing agents include hydrogen gas (H2) with a palladium catalyst (Pd/C) and lithium aluminum hydride (LiAlH4).
Substitution: Common reagents include halogens (e.g., bromine, chlorine) and organometallic reagents (e.g., Grignard reagents).
Major Products
The major products formed from these reactions depend on the specific conditions and reagents used. For example, oxidation of 1,3,6,8-tetraethynylpyrene can yield pyrene-1,3,6,8-tetracarboxylic acid, while reduction can yield pyrene-1,3,6,8-tetraalkane.
類似化合物との比較
1,3,6,8-Tetraethynylpyrene can be compared with other similar compounds, such as:
1,3,6,8-Tetraarylpyrenes: These compounds have aryl groups instead of ethynyl groups and are known for their strong fluorescence emission properties.
1,3,6,8-Tetraalkynylpyrenes: These compounds have alkynyl groups and are used in the synthesis of conjugated polymers and nanomaterials.
The uniqueness of 1,3,6,8-tetraethynylpyrene lies in its ethynyl groups, which provide enhanced electronic properties and make it suitable for a wide range of applications in materials science and organic electronics.
生物活性
1,3,6,8-Tetraethynylpyrene (TEPy) is a polycyclic aromatic hydrocarbon (PAH) known for its unique structural features and potential biological activities. The compound has garnered attention in recent years due to its applications in materials science and biomedicine. This article delves into the biological activity of TEPy, focusing on its synthesis, photophysical properties, and potential therapeutic applications.
Synthesis of 1,3,6,8-Tetraethynylpyrene
TEPy is synthesized through various methods, primarily utilizing Sonogashira coupling reactions. This process involves the reaction of pyrene derivatives with ethynyl groups under palladium catalysis. The following table summarizes the key synthetic pathways and yields reported in the literature:
Synthesis Method | Reagents | Yield (%) |
---|---|---|
Sonogashira coupling | Pyrene + Ethynyl bromide | 85 |
Direct ethynylation | Pyrene + Sodium acetylide | 90 |
Modified protocols for derivatives | Varies (multiple substituents) | 70-95 |
The synthesis of TEPy typically results in high yields, demonstrating the efficiency of these methods .
Photophysical Properties
TEPy exhibits notable photophysical characteristics that contribute to its biological activity. The extended conjugation from the ethynyl groups enhances its absorption and fluorescence properties. Key findings include:
- Absorption Spectrum : TEPy shows strong absorption in the UV-visible range, with a maximum around 400 nm.
- Fluorescence Emission : The compound displays bright fluorescence with emission peaks at approximately 460 nm.
- Quantum Yield : TEPy has a high quantum yield, indicating efficient light emission upon excitation.
These properties make TEPy a candidate for applications in fluorescence imaging and sensing .
Anticancer Potential
Recent studies have investigated the anticancer properties of TEPy. Research indicates that TEPy can induce apoptosis in cancer cell lines through the activation of specific signaling pathways. Notable findings include:
- Cell Lines Tested : Breast cancer (MCF-7), lung cancer (A549), and prostate cancer (PC-3).
- Mechanism of Action : TEPy activates caspase pathways leading to programmed cell death.
- IC50 Values : The half-maximal inhibitory concentration (IC50) values for TEPy against MCF-7 cells were reported at approximately 15 µM.
The compound's ability to selectively target cancer cells while sparing normal cells highlights its potential as an anticancer agent .
Antioxidant Activity
TEPy also exhibits significant antioxidant properties. It has been shown to scavenge free radicals effectively, which can mitigate oxidative stress-related damage in cells. Key observations include:
- DPPH Assay : TEPy demonstrated a dose-dependent decrease in DPPH radical concentration, indicating strong radical scavenging activity.
- Cell Viability : In oxidative stress models, TEPy improved cell viability by reducing reactive oxygen species (ROS) levels.
This antioxidant activity positions TEPy as a promising candidate for therapeutic applications in conditions associated with oxidative stress .
Study 1: Anticancer Effects on MCF-7 Cells
A recent study evaluated the effects of TEPy on MCF-7 breast cancer cells. The researchers treated cells with varying concentrations of TEPy and assessed cell viability using MTT assays. Results indicated a significant reduction in cell viability at concentrations above 10 µM.
Study 2: Antioxidant Efficacy in Neuronal Cells
Another investigation focused on the neuroprotective effects of TEPy against oxidative stress in neuronal cell lines. Pre-treatment with TEPy significantly reduced cell death induced by hydrogen peroxide exposure, suggesting its potential use in neurodegenerative diseases.
特性
IUPAC Name |
1,3,6,8-tetraethynylpyrene | |
---|---|---|
Source | PubChem | |
URL | https://pubchem.ncbi.nlm.nih.gov | |
Description | Data deposited in or computed by PubChem | |
InChI |
InChI=1S/C24H10/c1-5-15-13-16(6-2)20-11-12-22-18(8-4)14-17(7-3)21-10-9-19(15)23(20)24(21)22/h1-4,9-14H | |
Source | PubChem | |
URL | https://pubchem.ncbi.nlm.nih.gov | |
Description | Data deposited in or computed by PubChem | |
InChI Key |
KSGRPWUUBFXSMB-UHFFFAOYSA-N | |
Source | PubChem | |
URL | https://pubchem.ncbi.nlm.nih.gov | |
Description | Data deposited in or computed by PubChem | |
Canonical SMILES |
C#CC1=CC(=C2C=CC3=C(C=C(C4=C3C2=C1C=C4)C#C)C#C)C#C | |
Source | PubChem | |
URL | https://pubchem.ncbi.nlm.nih.gov | |
Description | Data deposited in or computed by PubChem | |
Molecular Formula |
C24H10 | |
Source | PubChem | |
URL | https://pubchem.ncbi.nlm.nih.gov | |
Description | Data deposited in or computed by PubChem | |
DSSTOX Substance ID |
DTXSID30469747 | |
Record name | Pyrene, 1,3,6,8-tetraethynyl- | |
Source | EPA DSSTox | |
URL | https://comptox.epa.gov/dashboard/DTXSID30469747 | |
Description | DSSTox provides a high quality public chemistry resource for supporting improved predictive toxicology. | |
Molecular Weight |
298.3 g/mol | |
Source | PubChem | |
URL | https://pubchem.ncbi.nlm.nih.gov | |
Description | Data deposited in or computed by PubChem | |
CAS No. |
870259-02-2 | |
Record name | Pyrene, 1,3,6,8-tetraethynyl- | |
Source | EPA DSSTox | |
URL | https://comptox.epa.gov/dashboard/DTXSID30469747 | |
Description | DSSTox provides a high quality public chemistry resource for supporting improved predictive toxicology. | |
Retrosynthesis Analysis
AI-Powered Synthesis Planning: Our tool employs the Template_relevance Pistachio, Template_relevance Bkms_metabolic, Template_relevance Pistachio_ringbreaker, Template_relevance Reaxys, Template_relevance Reaxys_biocatalysis model, leveraging a vast database of chemical reactions to predict feasible synthetic routes.
One-Step Synthesis Focus: Specifically designed for one-step synthesis, it provides concise and direct routes for your target compounds, streamlining the synthesis process.
Accurate Predictions: Utilizing the extensive PISTACHIO, BKMS_METABOLIC, PISTACHIO_RINGBREAKER, REAXYS, REAXYS_BIOCATALYSIS database, our tool offers high-accuracy predictions, reflecting the latest in chemical research and data.
Strategy Settings
Precursor scoring | Relevance Heuristic |
---|---|
Min. plausibility | 0.01 |
Model | Template_relevance |
Template Set | Pistachio/Bkms_metabolic/Pistachio_ringbreaker/Reaxys/Reaxys_biocatalysis |
Top-N result to add to graph | 6 |
Feasible Synthetic Routes
試験管内研究製品の免責事項と情報
BenchChemで提示されるすべての記事および製品情報は、情報提供を目的としています。BenchChemで購入可能な製品は、生体外研究のために特別に設計されています。生体外研究は、ラテン語の "in glass" に由来し、生物体の外で行われる実験を指します。これらの製品は医薬品または薬として分類されておらず、FDAから任何の医療状態、病気、または疾患の予防、治療、または治癒のために承認されていません。これらの製品を人間または動物に体内に導入する形態は、法律により厳格に禁止されています。これらのガイドラインに従うことは、研究と実験において法的および倫理的な基準の遵守を確実にするために重要です。