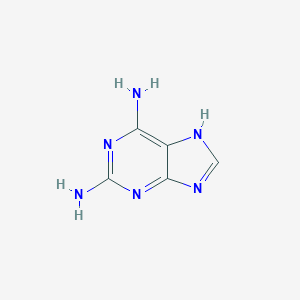
2,6-ジアミノプリン
概要
説明
2,6-Diaminopurine is a purine derivative with the chemical formula C₅H₆N₆. It is structurally similar to adenine, one of the four nucleobases in the nucleic acids of DNA and RNA. This compound is known for its ability to form three hydrogen bonds with thymine, enhancing the stability of nucleic acid duplexes. Historically, 2,6-diaminopurine has been used in the treatment of leukemia and is found in the genetic material of some bacteriophage viruses .
科学的研究の応用
2,6-Diaminopurine has a wide range of applications in scientific research:
Chemistry: It is used to study the structural properties of nucleic acids and their interactions with ligands.
Biology: It serves as a tool to investigate the stability and conformation of DNA and RNA duplexes.
Industry: It is used in the synthesis of oligonucleotides and other nucleic acid derivatives.
作用機序
Target of Action
2,6-Diaminopurine (also known as 2-aminoadenine) is an analogue of adenine and primarily targets the genetic material of cells . It is used instead of adenine in the genetic material of some bacteriophage viruses . It has also been used in the treatment of leukemia .
Mode of Action
2,6-Diaminopurine interacts with its targets by pairing with thymidine (or uracil), engaging in three hydrogen bonds of the Watson-Crick type . This interaction adds considerable stability to the double helix and imparts other structural features, such as an altered groove width and disruption of the normal spine of hydration .
Biochemical Pathways
The Z base (2,6-Diaminopurine) is produced by a pathway involving DUF550 (MazZ) and PurZ . The PrimPol/AEP DNA polymerase responsible for handling the Z base occurs in the same gene cluster as the three aforementioned enzymes . This improved stability affects protein-binding interactions that rely on those differences .
Pharmacokinetics
2,6-Diaminopurine has shown to be very stable in plasma and is distributed throughout the body . It can correct a nonsense mutation in the Cftr gene in vivo in a new CF mouse model, in utero, and through breastfeeding, thanks to its adequate pharmacokinetic properties .
Result of Action
The 2,6-Diaminopurine-Thymine pair affects the local flexibility of DNA and impedes the interaction with helix-bending proteins . By providing a non-canonical hydrogen bond donor in the minor groove and/or blocking access to the floor of that groove, it strongly affects interactions with small molecules such as antibiotics and anticancer drugs .
Action Environment
Environmental factors can influence the action of 2,6-Diaminopurine. For instance, it has been found in extracts of the edible mushroom Lepista inversa . It is thought to act as an inhibitor of a tRNA-specific 2’-O-methyltransferase and promotes the interaction of the near-cognate tRNA Trp with the UGA stop codon . This shows that the environment in which 2,6-Diaminopurine is found can influence its action, efficacy, and stability.
生化学分析
Biochemical Properties
2,6-Diaminopurine has been shown to have excellent electron-donating properties in nucleic acid strands . It spontaneously base pairs with uracil in water and the solid state without the need to be attached to the ribose-phosphate backbone . Depending on the reaction conditions, 2,6-Diaminopurine and uracil assemble in thermodynamically stable hydrated and anhydrated base-paired cocrystals .
Cellular Effects
In Calu-6 cancer cells, where the TP53 gene has a UGA nonsense mutation, 2,6-Diaminopurine treatment increases the p53 level . It also decreases the growth of tumors arising from Calu-6 cells injected into immunodeficient nude mice .
Molecular Mechanism
2,6-Diaminopurine enables repair of cyclobutane pyrimidine dimers (CPDs) with yields reaching 92% . This substantial self-repairing activity originates from the excellent electron-donating properties of 2,6-Diaminopurine in nucleic acid strands .
Temporal Effects in Laboratory Settings
Under UV irradiation, an aqueous solution of 2,6-Diaminopurine base pair undergoes photochemical degradation . 2,6-Diaminopurine and its 2′-deoxyribonucleoside are significantly photostable to UV radiation .
Metabolic Pathways
It has been suggested that 2,6-Diaminopurine could have played critical roles in the formation of functional and photostable RNA/DNA oligomers in UV-rich prebiotic environments .
Transport and Distribution
It has been shown that 2,6-Diaminopurine can spontaneously base pair with uracil in water and the solid state , suggesting it may be distributed within cells via base pairing interactions.
Subcellular Localization
Given its role in base pairing with uracil, it is likely to be found wherever RNA and DNA are located within the cell .
準備方法
Synthetic Routes and Reaction Conditions: 2,6-Diaminopurine can be synthesized through various methods. One common approach involves the reaction of guanine with ammonia under high pressure and temperature. Another method includes the use of halogenated purines such as 6-chloro-2-aminopurine, which undergoes nucleophilic substitution with ammonia .
Industrial Production Methods: Industrial production of 2,6-diaminopurine often involves multi-step synthetic processes. These processes typically start with commercially available purine derivatives, followed by protection and deprotection strategies to ensure the selective introduction of amino groups at the desired positions .
化学反応の分析
Types of Reactions: 2,6-Diaminopurine undergoes various chemical reactions, including:
Substitution Reactions: It can react with halogenated compounds to form substituted purines.
Oxidation and Reduction Reactions: These reactions can modify the functional groups attached to the purine ring.
Photochemical Reactions: Under UV irradiation, 2,6-diaminopurine can undergo photodegradation.
Common Reagents and Conditions:
Nucleophilic Substitution: Ammonia or amines are commonly used as nucleophiles.
Oxidation: Oxidizing agents such as hydrogen peroxide can be used.
Photochemical Conditions: UV light is used to induce photochemical reactions.
Major Products:
Substituted Purines: Products depend on the nature of the substituents introduced.
Oxidized or Reduced Purines: These products result from changes in the oxidation state of the purine ring.
類似化合物との比較
Adenine: Structurally similar but forms only two hydrogen bonds with thymine.
2-Aminoadenine: Another purine derivative with similar properties.
Guanine: Another nucleobase that forms three hydrogen bonds but pairs with cytosine.
Uniqueness: 2,6-Diaminopurine is unique due to its ability to form three hydrogen bonds with thymine, enhancing the stability of nucleic acid duplexes. This property makes it particularly useful in studies involving nucleic acid stability and interactions .
生物活性
2,6-Diaminopurine (DAP) is a purine derivative that has garnered attention for its significant biological activities, particularly in the realms of genetic mutation correction and DNA repair. This article synthesizes recent research findings, case studies, and data tables to provide a comprehensive overview of DAP's biological activity.
Overview of 2,6-Diaminopurine
2,6-Diaminopurine is structurally similar to adenine but possesses two amino groups at the 2 and 6 positions. This modification enhances its ability to interact with nucleic acids and has implications for its role in various biological processes.
1. DNA Repair Mechanisms
Recent studies have highlighted DAP's capacity to facilitate the repair of DNA lesions, particularly cyclobutane pyrimidine dimers (CPDs), which are common forms of UV-induced DNA damage. Research indicates that substituting adenine with DAP can yield repair efficiencies as high as 92% for CPDs. This remarkable self-repairing activity is attributed to DAP's excellent electron-donating properties within nucleic acid strands .
Table 1: Repair Efficiency of CPDs Using DAP
Nucleobase | Repair Efficiency (%) |
---|---|
Adenine | 50 |
2,6-Diaminopurine | 92 |
2. Correction of Nonsense Mutations
DAP has been identified as a potent suppressor of UGA premature stop codons. In a study involving cystic fibrosis (CF) mouse models, DAP was shown to correct nonsense mutations in the CFTR gene effectively. The compound demonstrated stability in plasma and favorable pharmacokinetics, allowing it to diffuse throughout the body and restore CFTR function in various tissues .
Case Study: Cystic Fibrosis Mouse Model
- Objective : Assess the efficacy of DAP in correcting CFTR gene mutations.
- Method : Daily administration of DAP in a CF mouse model.
- Results : Significant restoration of CFTR expression observed in lung and intestinal tissues.
3. Antimetabolite Properties
As an antimetabolite, DAP has potential applications in cancer therapy. It can interfere with nucleic acid synthesis, thus inhibiting cancer cell proliferation. Studies have shown that DAP can enhance the efficacy of other chemotherapeutic agents by increasing their uptake and effectiveness against neoplastic cells .
Table 2: Antimetabolite Effects of DAP
Treatment Combination | Observed Effect |
---|---|
DAP + Gemcitabine | Enhanced cytotoxicity |
DAP + Methotrexate | Synergistic effects on cell cycle arrest |
Mechanistic Insights
The mechanisms underlying DAP's biological activities involve its interaction with DNA and RNA structures:
- Electron Donation : The presence of amino groups enhances electron donation capabilities, facilitating repair processes.
- Base Pair Stability : Substitution of adenine with DAP increases base-pairing stability, which is crucial for maintaining genetic fidelity during replication and repair .
Future Directions
The promising biological activities of 2,6-diaminopurine suggest several avenues for future research:
- Therapeutic Applications : Further exploration into its use as a therapeutic agent for genetic disorders caused by nonsense mutations.
- Combination Therapies : Investigating its potential in combination with existing chemotherapeutic agents to enhance treatment efficacy.
- Prebiotic Chemistry : Understanding its role in prebiotic conditions may provide insights into the origins of life on Earth.
特性
IUPAC Name |
7H-purine-2,6-diamine | |
---|---|---|
Source | PubChem | |
URL | https://pubchem.ncbi.nlm.nih.gov | |
Description | Data deposited in or computed by PubChem | |
InChI |
InChI=1S/C5H6N6/c6-3-2-4(9-1-8-2)11-5(7)10-3/h1H,(H5,6,7,8,9,10,11) | |
Source | PubChem | |
URL | https://pubchem.ncbi.nlm.nih.gov | |
Description | Data deposited in or computed by PubChem | |
InChI Key |
MSSXOMSJDRHRMC-UHFFFAOYSA-N | |
Source | PubChem | |
URL | https://pubchem.ncbi.nlm.nih.gov | |
Description | Data deposited in or computed by PubChem | |
Canonical SMILES |
C1=NC2=NC(=NC(=C2N1)N)N | |
Source | PubChem | |
URL | https://pubchem.ncbi.nlm.nih.gov | |
Description | Data deposited in or computed by PubChem | |
Molecular Formula |
C5H6N6 | |
Source | PubChem | |
URL | https://pubchem.ncbi.nlm.nih.gov | |
Description | Data deposited in or computed by PubChem | |
Related CAS |
7280-83-3 (sulfate), 22194-11-2 (lactate salt/solvate) | |
Record name | 2,6-Diaminopurine | |
Source | ChemIDplus | |
URL | https://pubchem.ncbi.nlm.nih.gov/substance/?source=chemidplus&sourceid=0001904989 | |
Description | ChemIDplus is a free, web search system that provides access to the structure and nomenclature authority files used for the identification of chemical substances cited in National Library of Medicine (NLM) databases, including the TOXNET system. | |
DSSTOX Substance ID |
DTXSID0062052 | |
Record name | 2,6-Diaminopurine | |
Source | EPA DSSTox | |
URL | https://comptox.epa.gov/dashboard/DTXSID0062052 | |
Description | DSSTox provides a high quality public chemistry resource for supporting improved predictive toxicology. | |
Molecular Weight |
150.14 g/mol | |
Source | PubChem | |
URL | https://pubchem.ncbi.nlm.nih.gov | |
Description | Data deposited in or computed by PubChem | |
CAS No. |
1904-98-9, 133762-79-5 | |
Record name | 2,6-Diaminopurine | |
Source | CAS Common Chemistry | |
URL | https://commonchemistry.cas.org/detail?cas_rn=1904-98-9 | |
Description | CAS Common Chemistry is an open community resource for accessing chemical information. Nearly 500,000 chemical substances from CAS REGISTRY cover areas of community interest, including common and frequently regulated chemicals, and those relevant to high school and undergraduate chemistry classes. This chemical information, curated by our expert scientists, is provided in alignment with our mission as a division of the American Chemical Society. | |
Explanation | The data from CAS Common Chemistry is provided under a CC-BY-NC 4.0 license, unless otherwise stated. | |
Record name | 2,6-Diaminopurine | |
Source | ChemIDplus | |
URL | https://pubchem.ncbi.nlm.nih.gov/substance/?source=chemidplus&sourceid=0001904989 | |
Description | ChemIDplus is a free, web search system that provides access to the structure and nomenclature authority files used for the identification of chemical substances cited in National Library of Medicine (NLM) databases, including the TOXNET system. | |
Record name | 2,6-Diamino-purin-9-yl | |
Source | ChemIDplus | |
URL | https://pubchem.ncbi.nlm.nih.gov/substance/?source=chemidplus&sourceid=0133762795 | |
Description | ChemIDplus is a free, web search system that provides access to the structure and nomenclature authority files used for the identification of chemical substances cited in National Library of Medicine (NLM) databases, including the TOXNET system. | |
Record name | 2,6-Diaminopurine | |
Source | DTP/NCI | |
URL | https://dtp.cancer.gov/dtpstandard/servlet/dwindex?searchtype=NSC&outputformat=html&searchlist=743 | |
Description | The NCI Development Therapeutics Program (DTP) provides services and resources to the academic and private-sector research communities worldwide to facilitate the discovery and development of new cancer therapeutic agents. | |
Explanation | Unless otherwise indicated, all text within NCI products is free of copyright and may be reused without our permission. Credit the National Cancer Institute as the source. | |
Record name | 9H-Purine-2,6-diamine | |
Source | EPA Chemicals under the TSCA | |
URL | https://www.epa.gov/chemicals-under-tsca | |
Description | EPA Chemicals under the Toxic Substances Control Act (TSCA) collection contains information on chemicals and their regulations under TSCA, including non-confidential content from the TSCA Chemical Substance Inventory and Chemical Data Reporting. | |
Record name | 2,6-Diaminopurine | |
Source | EPA DSSTox | |
URL | https://comptox.epa.gov/dashboard/DTXSID0062052 | |
Description | DSSTox provides a high quality public chemistry resource for supporting improved predictive toxicology. | |
Record name | Purine-2,6-diyldiamine | |
Source | European Chemicals Agency (ECHA) | |
URL | https://echa.europa.eu/substance-information/-/substanceinfo/100.016.006 | |
Description | The European Chemicals Agency (ECHA) is an agency of the European Union which is the driving force among regulatory authorities in implementing the EU's groundbreaking chemicals legislation for the benefit of human health and the environment as well as for innovation and competitiveness. | |
Explanation | Use of the information, documents and data from the ECHA website is subject to the terms and conditions of this Legal Notice, and subject to other binding limitations provided for under applicable law, the information, documents and data made available on the ECHA website may be reproduced, distributed and/or used, totally or in part, for non-commercial purposes provided that ECHA is acknowledged as the source: "Source: European Chemicals Agency, http://echa.europa.eu/". Such acknowledgement must be included in each copy of the material. ECHA permits and encourages organisations and individuals to create links to the ECHA website under the following cumulative conditions: Links can only be made to webpages that provide a link to the Legal Notice page. | |
Record name | 2,6-DIAMINOPURINE | |
Source | FDA Global Substance Registration System (GSRS) | |
URL | https://gsrs.ncats.nih.gov/ginas/app/beta/substances/49P95BAU4Z | |
Description | The FDA Global Substance Registration System (GSRS) enables the efficient and accurate exchange of information on what substances are in regulated products. Instead of relying on names, which vary across regulatory domains, countries, and regions, the GSRS knowledge base makes it possible for substances to be defined by standardized, scientific descriptions. | |
Explanation | Unless otherwise noted, the contents of the FDA website (www.fda.gov), both text and graphics, are not copyrighted. They are in the public domain and may be republished, reprinted and otherwise used freely by anyone without the need to obtain permission from FDA. Credit to the U.S. Food and Drug Administration as the source is appreciated but not required. | |
Synthesis routes and methods I
Procedure details
Synthesis routes and methods II
Procedure details
Synthesis routes and methods III
Procedure details
Retrosynthesis Analysis
AI-Powered Synthesis Planning: Our tool employs the Template_relevance Pistachio, Template_relevance Bkms_metabolic, Template_relevance Pistachio_ringbreaker, Template_relevance Reaxys, Template_relevance Reaxys_biocatalysis model, leveraging a vast database of chemical reactions to predict feasible synthetic routes.
One-Step Synthesis Focus: Specifically designed for one-step synthesis, it provides concise and direct routes for your target compounds, streamlining the synthesis process.
Accurate Predictions: Utilizing the extensive PISTACHIO, BKMS_METABOLIC, PISTACHIO_RINGBREAKER, REAXYS, REAXYS_BIOCATALYSIS database, our tool offers high-accuracy predictions, reflecting the latest in chemical research and data.
Strategy Settings
Precursor scoring | Relevance Heuristic |
---|---|
Min. plausibility | 0.01 |
Model | Template_relevance |
Template Set | Pistachio/Bkms_metabolic/Pistachio_ringbreaker/Reaxys/Reaxys_biocatalysis |
Top-N result to add to graph | 6 |
Feasible Synthetic Routes
Q1: How does 2,6-diaminopurine exert its effects in biological systems?
A1: 2,6-diaminopurine primarily acts as an adenine analog and can be incorporated into DNA during replication. [, ] This incorporation can disrupt DNA synthesis and function, leading to growth inhibition in various cell types, including tumor cells. [, ] Additionally, 2,6-diaminopurine can be metabolized to 2,6-diaminopurine riboside and subsequently phosphorylated to its triphosphate form, potentially interfering with nucleotide metabolism. [, ]
Q2: How does the presence of an additional amino group at position 2 of 2,6-diaminopurine affect its interaction with nucleic acids compared to adenine?
A2: The additional amino group allows 2,6-diaminopurine to form three hydrogen bonds with uracil, leading to increased stability of oligonucleotide duplexes compared to adenine, which forms only two hydrogen bonds. [, ] This property makes 2,6-diaminopurine a valuable tool in nucleic acid research and drug development. [, , ]
Q3: What are the implications of 2,6-diaminopurine's conversion to deoxyguanosine for its mechanism of action?
A3: In certain cell lines, like L1210 cells, 2,6-diaminopurine can act as a prodrug for deoxyguanosine due to efficient conversion by adenosine deaminase. [] This conversion leads to a marked increase in dGTP levels, which in turn inhibits DNA synthesis and ribonucleotide reductase activity, contributing to the compound's antiproliferative effect. []
Q4: What is the molecular formula and weight of 2,6-diaminopurine?
A4: The molecular formula of 2,6-diaminopurine is C5H6N6, and its molecular weight is 150.14 g/mol.
Q5: Are there any characteristic spectroscopic data available for 2,6-diaminopurine?
A5: While specific spectroscopic data is not extensively detailed within the provided research, UV absorbance is a common method for studying 2,6-diaminopurine and its derivatives. [, , ] Additionally, NMR spectroscopy, particularly 1H NMR, has been used to analyze the structure and isomeric configurations of 2,6-diaminopurine derivatives. [, , ]
Q6: How do structural modifications to the 2,6-diaminopurine scaffold affect its biological activity?
A6: Modifications to the 2,6-diaminopurine scaffold, particularly at the N9 and C2/C6 positions, significantly influence its activity and target selectivity. [, ] For instance, introducing a cyclopropyl group at the N6 position of 9-(2-phosphonylmethoxyethyl)-2,6-diaminopurine (PMEDAP) led to the creation of cPr-PMEDAP, which demonstrated superior antitumor efficacy and selectivity compared to the parent compound in a rat choriocarcinoma model. [] Similarly, substituting adenine with 2,6-diaminopurine in oligonucleotide N3′→P5′ phosphoramidates resulted in a marked stabilization of complexes formed with complementary DNA and RNA strands, highlighting the importance of the additional amino group for binding affinity. [] Conversely, replacing guanosine with inosine in DNA sequences, which effectively removes a 2-amino group, can significantly hinder the binding of molecules like actinomycin D, emphasizing the crucial role of the 2-amino group in molecular recognition. []
Q7: What is the impact of sugar modifications on the activity of 2,6-diaminopurine nucleosides?
A7: Sugar modifications significantly influence the antiviral activity of 2,6-diaminopurine nucleosides. [, , ] For example, the 3′-azido and 3′-fluoro derivatives of 2,6-diaminopurine-2′,3′-dideoxyriboside exhibited potent anti-HIV activity, highlighting the importance of the 3′-substituent for antiviral efficacy. [] Conversely, the 9-β-D-arabinoside and 9-β-D-2′-deoxyxyloside derivatives lacked antiretroviral activity, indicating that specific sugar modifications are crucial for targeting HIV. [] Furthermore, the introduction of a 4′-C-ethynyl group in 2′-deoxypurine nucleosides resulted in potent anti-HIV activity, but also increased toxicity. [] These findings emphasize the need for careful consideration of sugar modifications to balance antiviral activity with acceptable toxicity profiles.
Q8: What analytical methods are commonly used to study 2,6-diaminopurine and its derivatives?
A8: Several analytical techniques are employed to study 2,6-diaminopurine and its derivatives. These include:
- Chromatographic techniques: Ion-exchange chromatography has been used to separate and identify acid-soluble metabolites of 2,6-diaminopurine. [] High-performance liquid chromatography (HPLC) is likely utilized for the analysis and purification of synthesized compounds, although not explicitly mentioned in the provided abstracts.
- Spectroscopic techniques: UV-Vis spectroscopy is frequently used to assess the binding of 2,6-diaminopurine-containing oligonucleotides to their complementary strands by measuring changes in absorbance upon complex formation. [, ] NMR spectroscopy, particularly 1H NMR, has been employed to confirm the structures of synthesized 2,6-diaminopurine derivatives and to analyze their isomeric configurations. [, , ]
- Electrochemical techniques: Cyclic voltammetry has been used to study the electrochemical oxidation of 2,6-diaminopurine on modified electrodes, providing insights into its electron transfer properties and potential for electrochemical sensing applications. []
試験管内研究製品の免責事項と情報
BenchChemで提示されるすべての記事および製品情報は、情報提供を目的としています。BenchChemで購入可能な製品は、生体外研究のために特別に設計されています。生体外研究は、ラテン語の "in glass" に由来し、生物体の外で行われる実験を指します。これらの製品は医薬品または薬として分類されておらず、FDAから任何の医療状態、病気、または疾患の予防、治療、または治癒のために承認されていません。これらの製品を人間または動物に体内に導入する形態は、法律により厳格に禁止されています。これらのガイドラインに従うことは、研究と実験において法的および倫理的な基準の遵守を確実にするために重要です。