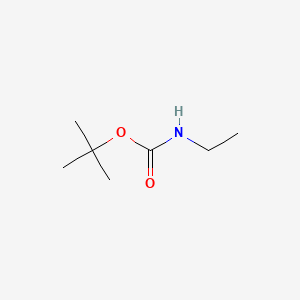
Tert-butyl N-ethylcarbamate
概要
説明
Tert-butyl N-ethylcarbamate is a carbamate derivative characterized by a tert-butoxycarbonyl (Boc) group attached to an ethylamine moiety. Its general structure is tert-butyl-O-C(=O)-NH-CH2CH3. This compound serves as a key intermediate in organic synthesis, particularly in peptide chemistry and drug design, where the Boc group acts as a protective moiety for amines .
作用機序
Target of Action
Tert-butyl ethylcarbamate, also known as Tert-butyl N-ethylcarbamate or tert-butylethylcarbamate, is a type of carbamate compound . Carbamates are known to interact with various targets in the body, including enzymes, receptors, and other proteins . .
Mode of Action
Carbamates in general are known to interact with their targets by forming covalent bonds, leading to changes in the target’s function
Biochemical Pathways
Carbamates have been implicated in the dysregulation of the immune system, potentially affecting various immune-related pathways . They may initiate, facilitate, or exacerbate pathological immune processes, resulting in immunotoxicity .
Result of Action
Carbamates have been implicated in the dysregulation of the immune system, potentially leading to diseases associated with alterations of the immune response, such as hypersensitivity reactions, some autoimmune diseases, and cancers .
生物活性
Tert-butyl N-ethylcarbamate (TBNEC) is a carbamate derivative with potential biological activities that merit detailed exploration. This article synthesizes available research findings, case studies, and data on the biological activity of TBNEC, emphasizing its pharmacological properties and mechanisms of action.
Chemical Structure and Properties
TBNEC has the chemical formula C₇H₁₅NO₂ and is classified under carbamates, which are known for their diverse biological activities. The compound's structure features a tert-butyl group attached to an ethyl carbamate moiety, influencing its solubility and reactivity.
Biological Activity Overview
Research indicates that TBNEC exhibits various biological activities, including:
Case Study 1: Enzyme Inhibition
A study focused on the design and synthesis of dipeptide-type inhibitors reported that compounds structurally similar to TBNEC exhibited significant inhibitory activity against the SARS-CoV 3CL protease. The IC₅₀ values for these inhibitors were determined through fluorometric assays, revealing promising results for further development as antiviral agents .
Case Study 2: Cytotoxicity Evaluation
In a cytotoxicity assessment involving HepG2 cells, TBNEC was tested alongside various oligopeptides. The results indicated that certain concentrations led to a notable reduction in cell viability, suggesting that TBNEC may influence cellular pathways associated with apoptosis or necrosis .
Table 1: Summary of Biological Activities of TBNEC
The biological activity of TBNEC is hypothesized to stem from its ability to interact with specific protein targets within cells. For example:
- Protease Inhibition : The mechanism involves binding to the active site of proteases, preventing substrate cleavage and subsequent viral replication.
- Cellular Pathways : The observed cytotoxic effects may be mediated through pathways involving oxidative stress or disruption of cellular homeostasis.
科学的研究の応用
Medicinal Chemistry
TBEC serves as an intermediate in the synthesis of pharmaceutical compounds. Its structure allows it to interact with specific enzymes and receptors, making it valuable in drug development. For instance, TBEC derivatives have been studied for their potential to inhibit certain biological pathways relevant to disease treatment.
Biological Studies
Research indicates that TBEC exhibits biological activity , including:
- Antimicrobial Properties: Studies have shown that TBEC can inhibit the growth of various microbial strains.
- Anticancer Activity: Preliminary investigations suggest that TBEC may induce apoptosis in cancer cells, making it a candidate for further pharmacological studies.
Industrial Applications
In the industrial sector, TBEC is utilized in:
- Agrochemicals Production: Its unique chemical properties allow for the development of herbicides and pesticides.
- Specialty Chemicals: TBEC is used as a building block for synthesizing complex organic molecules, enhancing its utility in various chemical processes.
Case Studies
Several case studies highlight the applications of TBEC:
-
Antimicrobial Activity Study:
- Researchers tested TBEC against various bacterial strains, demonstrating significant inhibitory effects comparable to standard antibiotics.
-
Cancer Cell Line Testing:
- In vitro studies showed that TBEC induced apoptosis in breast cancer cell lines, leading to a reduction in cell viability.
-
Agrochemical Development:
- TBEC derivatives were formulated into a new herbicide that showed enhanced efficacy compared to existing products in field trials.
Q & A
Basic Questions
Q. What are the standard synthetic routes for tert-butyl N-ethylcarbamate, and how is its structural integrity confirmed?
The compound is typically synthesized via nucleophilic substitution between tert-butyl carbamate and ethylamine derivatives under basic conditions. Solvents like dichloromethane or tetrahydrofuran (THF) are used, with triethylamine as a base to deprotonate the amine. Structural confirmation relies on spectroscopic methods: 1H/13C NMR for verifying the tert-butyl and ethyl groups, IR spectroscopy for carbamate C=O and N-H stretches, and mass spectrometry (MS) for molecular weight validation .
Q. Which analytical techniques are recommended for assessing purity post-synthesis?
High-performance liquid chromatography (HPLC) or gas chromatography (GC) with UV/RI detection is used for purity analysis. Elemental analysis (EA) ensures stoichiometric consistency, while differential scanning calorimetry (DSC) identifies thermal degradation points. Residual solvents are quantified via headspace GC .
Q. What solvents and reaction conditions optimize the synthesis of this compound derivatives?
Polar aprotic solvents (e.g., THF, acetonitrile) enhance nucleophilicity. Reactions are conducted at 0–25°C to minimize side reactions. pH is maintained at 8–10 using sodium hydroxide or triethylamine to deprotonate the amine. Post-synthesis, column chromatography (silica gel, ethyl acetate/hexane) isolates the product .
Advanced Research Questions
Q. How do steric effects of the tert-butyl group influence reactivity in nucleophilic substitutions?
The tert-butyl group’s steric bulk hinders nucleophilic attack at the carbamate carbonyl, directing reactivity toward the ethylamine moiety. Computational studies (DFT) show increased activation energy for undesired pathways, favoring selective alkylation. This is critical in designing derivatives for enzyme inhibition studies .
Q. What experimental strategies resolve contradictions in reported biological activities across studies?
Conflicting results (e.g., enzyme inhibition vs. activation) may arise from assay conditions (pH, temperature) or impurity profiles. Orthogonal validation using isothermal titration calorimetry (ITC) and surface plasmon resonance (SPR) clarifies binding kinetics. Replicating studies under standardized protocols (e.g., fixed ionic strength) minimizes variability .
Q. How can reaction yields be optimized while avoiding carbamate degradation?
Yield optimization requires strict temperature control (e.g., <40°C to prevent tert-butyl cleavage) and inert atmospheres (N2/Ar) to limit oxidation. Catalytic additives like 4-dimethylaminopyridine (DMAP) accelerate reactions without side products. Post-reaction quenching with aqueous HCl preserves product integrity .
Q. What mechanistic insights explain this compound’s alkylation of biological targets?
The compound acts as an alkylating agent via SN2 mechanisms, targeting nucleophilic residues (e.g., cysteine thiols, lysine amines) in enzymes. Mass spectrometry identifies covalent adducts, while X-ray crystallography reveals binding site conformations. Comparative studies with non-ethyl derivatives highlight the ethyl group’s role in modulating reactivity .
Q. How do environmental factors (pH, temperature) impact compound stability in biological assays?
Stability studies using accelerated degradation protocols (e.g., 40°C, pH 3–10) show hydrolysis of the carbamate group under acidic conditions. Buffers like PBS (pH 7.4) or HEPES (pH 8.0) stabilize the compound during cell-based assays. LC-MS monitors degradation products like CO2 and tert-butanol .
Q. What structural modifications enhance selectivity toward specific biological targets?
Substituting the ethyl group with bulkier alkyl chains (e.g., isopropyl) reduces off-target interactions. Introducing electron-withdrawing groups (e.g., fluorine) on the carbamate nitrogen increases electrophilicity, improving binding to serine hydrolases. Structure-activity relationship (SAR) studies guide rational design .
Q. How are conflicting spectroscopic data (e.g., NMR shifts) reconciled during structural elucidation?
Discrepancies in NMR chemical shifts arise from solvent polarity or tautomerism. Low-temperature NMR (−40°C) suppresses dynamic effects, while 2D techniques (HSQC, HMBC) confirm connectivity. Cross-validation with X-ray crystallography or computational modeling (e.g., Gaussian) resolves ambiguities .
Q. Data Contradiction Analysis
Q. Why do some studies report this compound as a protease inhibitor, while others classify it as a substrate?
Context-dependent behavior stems from assay design. In high-concentration setups, the compound saturates active sites, mimicking inhibition. At lower concentrations, enzymatic hydrolysis dominates, classifying it as a substrate. Kinetic assays (Michaelis-Menten plots) differentiate these roles .
Q. How do solvent polarity and dielectric constant affect reaction outcomes in carbamate chemistry?
Polar solvents (ε > 20) stabilize transition states in SN2 mechanisms, favoring carbamate formation. Nonpolar solvents (ε < 5) promote elimination side reactions. Solvent screening via Design of Experiments (DoE) identifies optimal conditions for scalability .
Q. Methodological Guidelines
- For SAR Studies : Combine molecular docking (AutoDock Vina) with synthetic accessibility scores (SYNOPSIS) to prioritize derivatives .
- For Stability Testing : Use forced degradation studies (ICH Q1A guidelines) to predict shelf-life under accelerated conditions .
- For Data Reproducibility : Adopt FAIR data principles (Findable, Accessible, Interoperable, Reusable) in publishing spectral and assay data .
類似化合物との比較
Comparison with Structurally Related Carbamates
Structural Variations and Functional Group Impact
The tert-butyl carbamate family exhibits diversity through substitution at the nitrogen atom. Below is a comparative analysis of key derivatives:
Key Observations:
- Substituent Effects on Reactivity : Bulky groups (e.g., 4-chlorophenethyl in ) reduce hydrolysis rates, enhancing stability under acidic conditions. Hydrophilic substituents like -OH () improve aqueous solubility, making them suitable for prodrugs.
- Biological Activity : highlights that carbamates with ethyl or methyl groups (e.g., rivastigmine analogs) exhibit cholinesterase inhibition, with selectivity modulated by substituent size and polarity .
- Synthetic Utility : Derivatives with unsaturated groups (e.g., diallyl in ) are used in polymer crosslinking, while trifluoromethyl-containing analogs (e.g., ) show enhanced electronic properties for catalysis .
準備方法
Common Preparation Methods
Boc Protection of Ethylamine
The most straightforward and widely used method involves the reaction of ethylamine with di-tert-butyl dicarbonate under basic conditions:
Reaction:
Ethylamine + Di-tert-butyl dicarbonate → Tert-butyl N-ethylcarbamate + CO2-
- Solvent: Dichloromethane or tetrahydrofuran (THF)
- Base: Sodium bicarbonate or triethylamine to neutralize released acid
- Temperature: Room temperature
- Time: Several hours under inert atmosphere to avoid moisture
Purification:
Standard purification methods such as recrystallization or column chromatography are employed to isolate the product with high purity (>95%).
This method is favored for its simplicity and scalability, making it suitable for industrial production.
Multi-step Synthesis via N-tert-butyloxycarbonyl Intermediates
An alternative, more complex route involves multi-step synthesis starting from N-tert-butyloxycarbonyl-1,2-ethylenediamine or related intermediates:
Step 1: Reduction reaction of N-tert-butyloxycarbonyl-1,2-ethylenediamine with paraformaldehyde in an organic solvent (e.g., toluene or benzene) in the presence of acid (acetic acid or p-toluenesulfonic acid) at reflux to form 2-(N-isobutoxyformamido)ethyl isocyanide.
Step 2: Reduction of the isocyanide intermediate with sodium borohydride in an aprotic solvent (THF or dimethylformamide) at 20–30°C to afford this compound.
-
- Step 1: 4–6 hours
- Step 2: 3–5 hours
-
- Higher overall yield in two steps
- Lower cost of raw materials
- Reduced waste generation, suitable for industrial scale
-
- Requires careful control of reaction conditions
- Use of toxic reagents like sodium borohydride and isocyanide intermediates
This method is detailed in patent CN104086460B and is notable for industrial applicability.
Comparative Data Table of Preparation Methods
Detailed Research Findings and Notes
Reaction Mechanism Insights:
The Boc protection proceeds via nucleophilic attack of the amine on the electrophilic carbonyl carbon of di-tert-butyl dicarbonate, releasing CO2 and forming the carbamate bond.Control of Reaction Parameters:
The presence of base is crucial to neutralize acidic by-products and prevent Boc group hydrolysis. Temperature control avoids side reactions and decomposition.Purification Techniques:
Recrystallization from appropriate solvents or silica gel chromatography ensures removal of unreacted starting materials and side products.Industrial Considerations:
The two-step reduction method offers advantages in cost and environmental impact, making it attractive for large-scale synthesis despite its complexity.Safety and Environmental Notes: Handling of sodium borohydride and isocyanide intermediates requires strict safety protocols due to toxicity and reactivity.
特性
IUPAC Name |
tert-butyl N-ethylcarbamate | |
---|---|---|
Source | PubChem | |
URL | https://pubchem.ncbi.nlm.nih.gov | |
Description | Data deposited in or computed by PubChem | |
InChI |
InChI=1S/C7H15NO2/c1-5-8-6(9)10-7(2,3)4/h5H2,1-4H3,(H,8,9) | |
Source | PubChem | |
URL | https://pubchem.ncbi.nlm.nih.gov | |
Description | Data deposited in or computed by PubChem | |
InChI Key |
ISTGQSQWSKCNFJ-UHFFFAOYSA-N | |
Source | PubChem | |
URL | https://pubchem.ncbi.nlm.nih.gov | |
Description | Data deposited in or computed by PubChem | |
Canonical SMILES |
CCNC(=O)OC(C)(C)C | |
Source | PubChem | |
URL | https://pubchem.ncbi.nlm.nih.gov | |
Description | Data deposited in or computed by PubChem | |
Molecular Formula |
C7H15NO2 | |
Source | PubChem | |
URL | https://pubchem.ncbi.nlm.nih.gov | |
Description | Data deposited in or computed by PubChem | |
DSSTOX Substance ID |
DTXSID80458525 | |
Record name | Tert-butyl N-ethylcarbamate | |
Source | EPA DSSTox | |
URL | https://comptox.epa.gov/dashboard/DTXSID80458525 | |
Description | DSSTox provides a high quality public chemistry resource for supporting improved predictive toxicology. | |
Molecular Weight |
145.20 g/mol | |
Source | PubChem | |
URL | https://pubchem.ncbi.nlm.nih.gov | |
Description | Data deposited in or computed by PubChem | |
CAS No. |
38267-76-4 | |
Record name | Tert-butyl N-ethylcarbamate | |
Source | EPA DSSTox | |
URL | https://comptox.epa.gov/dashboard/DTXSID80458525 | |
Description | DSSTox provides a high quality public chemistry resource for supporting improved predictive toxicology. | |
Retrosynthesis Analysis
AI-Powered Synthesis Planning: Our tool employs the Template_relevance Pistachio, Template_relevance Bkms_metabolic, Template_relevance Pistachio_ringbreaker, Template_relevance Reaxys, Template_relevance Reaxys_biocatalysis model, leveraging a vast database of chemical reactions to predict feasible synthetic routes.
One-Step Synthesis Focus: Specifically designed for one-step synthesis, it provides concise and direct routes for your target compounds, streamlining the synthesis process.
Accurate Predictions: Utilizing the extensive PISTACHIO, BKMS_METABOLIC, PISTACHIO_RINGBREAKER, REAXYS, REAXYS_BIOCATALYSIS database, our tool offers high-accuracy predictions, reflecting the latest in chemical research and data.
Strategy Settings
Precursor scoring | Relevance Heuristic |
---|---|
Min. plausibility | 0.01 |
Model | Template_relevance |
Template Set | Pistachio/Bkms_metabolic/Pistachio_ringbreaker/Reaxys/Reaxys_biocatalysis |
Top-N result to add to graph | 6 |
Feasible Synthetic Routes
試験管内研究製品の免責事項と情報
BenchChemで提示されるすべての記事および製品情報は、情報提供を目的としています。BenchChemで購入可能な製品は、生体外研究のために特別に設計されています。生体外研究は、ラテン語の "in glass" に由来し、生物体の外で行われる実験を指します。これらの製品は医薬品または薬として分類されておらず、FDAから任何の医療状態、病気、または疾患の予防、治療、または治癒のために承認されていません。これらの製品を人間または動物に体内に導入する形態は、法律により厳格に禁止されています。これらのガイドラインに従うことは、研究と実験において法的および倫理的な基準の遵守を確実にするために重要です。