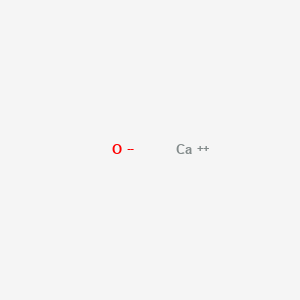
Calciumoxide
概要
説明
Calcium Oxide is a widely used chemical compound. It is a white, caustic, alkaline, crystalline solid at room temperature . The broadly used term lime connotes calcium-containing inorganic compounds, in which carbonates, oxides, and hydroxides of calcium, silicon, magnesium, aluminium, and iron predominate .
Synthesis Analysis
Calcium Oxide is usually made by the thermal decomposition of materials, such as limestone or seashells, that contain calcium carbonate (CaCO3; mineral calcite) in a lime kiln . This process is known as calcination. It starts with thermally decomposing the reactants at high temperatures but ensuring that the temperature is kept well below the melting point . Calcium carbonate undergoes calcination at temperatures ranging between 1070°C-1270°C .Molecular Structure Analysis
Calcium Oxide molecules contain one calcium cation (which holds a charge of +2) and one oxygen anion (which holds a charge of -2) . Thus, it can be understood that calcium oxide is an ionic compound featuring an ionic bond between calcium and oxygen . Solid calcium oxide possesses a halite, rock salt cubic crystal structure having the space group cF8 .Chemical Reactions Analysis
The primary chemical reaction involving Calcium Oxide is its reaction with water to form Calcium Hydroxide . This reaction is strongly exothermic and takes place vigorously with the formation of clouds of steam . The reaction can be represented as follows:CaO + H2O → Ca(OH)2
Physical And Chemical Properties Analysis
Calcium Oxide is an amorphous white solid with a high melting point of 2600°C . It is a very stable compound and withstands high temperatures . It has a medium viscosity and a high surface tension, plus a high to intermediate expansion and contraction rate . This material isn’t volatile at ceramic temperatures . Calcium Oxide has a moderate effect on color, except in large amounts when it may have a bleaching effect on iron oxide .科学的研究の応用
Catalytic Properties in Energy Applications
Calcium manganese oxides, including CaO, have been studied for their catalytic activities, particularly in addressing energy and environmental problems. The Ca–Mn–O systems exhibit potential in catalytic water oxidation and other energy-related applications due to their exceptional catalytic activities, inexpensive synthesis methods, and environmentally benign nature (Gagrani & Tsuzuki, 2019).
Interaction with Water
The hydration of calcium oxide surfaces has been explored using reactive force field molecular dynamics. This research is significant for understanding CaO's role in various applications like CO2 sequestration and biodiesel production, where surface hydroxylation is a key factor in catalytic performance (Manzano et al., 2012).
Biodiesel Production
Calcium oxide has been investigated for its accelerated catalytic activity in biodiesel production. The research focuses on optimizing the transesterification of plant oil with methanol, revealing that CaO can act effectively as a heterogeneous catalyst in this process (Kawashima, Matsubara, & Honda, 2009).
Carbon Dioxide Capture
Studies have reviewed calcium oxide's application in solar thermochemical carbon dioxide capture. This involves the assessment of thermodynamics, kinetics, and reactor design, emphasizing CaO's potential in environmental management (Reich et al., 2014).
In Steel Processing
Calcium oxide plays a role in steel processing, particularly in improving steel cleanliness and modifying inclusions for better quality steel. The formation of oxide-sulfide duplex inclusions and their avoidance during ladle treatment of liquid steel has been studied, highlighting CaO's significance in industrial metallurgy (Choudhary & Ghosh, 2008).
Non-Cement Structural Binder
Research has demonstrated the use of calcium oxide as an activator for ground granulated blast furnace slag, producing non-cement structural binders. This application of CaO can lead to significant advancements in construction materials and methods (Kim et al., 2013).
Dental Applications
Calcium oxide-based materials, like Biodentine, have gained popularity in dentistry due to their biologic properties and potential as a dentine replacement material. These materials are formulated using MTA-based cement technology, highlighting CaO's relevance in medical and dental applications (Sj et al., 2021).
CO2 Capture and Hydrogen Production
CaO nanoparticles have been enhanced for improved sorption performance, particularly in CO2 capture and hydrogen production. This research emphasizes the development of high-performance, low-cost catalysts, crucial for sustainable energy production (Islam et al., 2014).
Flotation in Mineral Processing
In the mineral processing industry, CaO is used as a pH modifier to promote the selective flotation of sulphide minerals. Its operational role and interaction with other chemicals during mineral flotation processes have been extensively reviewed (Zanin, Lambert, & Plessis, 2019).
Arsenic Co-Precipitation
Calcium oxide enhances ferric hydroxide co-precipitation of arsenic, especially in the presence of silicate. This application is significant for water treatment and environmental remediation, demonstrating CaO's effectiveness in removing hazardous elements from water (Ruiping et al., 2007).
Thermochemical Heat Storage
CaO-based materials are being explored for thermochemical heat storage in concentrated solar power plants. This research focuses on enhancing the cyclic hydration-dehydration performance of CaO, essential for energy storage applications (Sakellariou et al., 2015).
Gasification and Tar Reduction
Calcium oxide has been used in syngas conditioning to reduce tar production during the gasification of biomass. This application demonstrates CaO's potential in bioenergy production and environmental sustainability (Jordan & Akay, 2013).
Soil Decontamination
Nano-metallic Ca/CaO mixtures have been employed for simultaneous remediation of soils contaminated with heavy metals and PCBs. This innovative approach signifies CaO's utility in complex environmental cleanup operations (Mallampati et al., 2014).
Construction Material
The interaction of CaO with polycarboxylate ether-based superplasticizers affects the dissolution and hydration processes, vital for its use in construction as an expansive agent or repairing material (Kang et al., 2021).
Biodiesel Production Enhancement
Novel magnetic CaO-based catalysts have been developed to enhance the catalytic activity and stability of CaO in biodiesel production, addressing issues like Ca2+ leaching and providing efficient, reusable catalysts (Li et al., 2020).
Biodiesel from Waste Mussel Shells
Calcium oxide derived from waste mussel shells has been optimized for biodiesel production, showcasing an eco-friendly approach to catalyst production and recycling (Rezaei, Mohadesi, & Moradi, 2013).
CO2 Capture in Energy Processes
The operational window and efficiency penalty of CaO in CO2 capture, specifically in sorption-enhanced steam methane reforming processes, have been analyzed, highlighting its role in mitigating greenhouse gas emissions (Solieman et al., 2009).
作用機序
将来の方向性
Calcium Oxide nanoparticles have been synthesized using various methods and are being studied for their potential applications . For instance, they have been used in the synthesis of Calcium Oxide nanoparticles for CO2 capture . The future of Calcium Oxide lies in exploring its potential uses in various fields and improving the methods of its synthesis.
特性
IUPAC Name |
calcium;oxygen(2-) | |
---|---|---|
Source | PubChem | |
URL | https://pubchem.ncbi.nlm.nih.gov | |
Description | Data deposited in or computed by PubChem | |
InChI |
InChI=1S/Ca.O/q+2;-2 | |
Source | PubChem | |
URL | https://pubchem.ncbi.nlm.nih.gov | |
Description | Data deposited in or computed by PubChem | |
InChI Key |
BRPQOXSCLDDYGP-UHFFFAOYSA-N | |
Source | PubChem | |
URL | https://pubchem.ncbi.nlm.nih.gov | |
Description | Data deposited in or computed by PubChem | |
Canonical SMILES |
[O-2].[Ca+2] | |
Source | PubChem | |
URL | https://pubchem.ncbi.nlm.nih.gov | |
Description | Data deposited in or computed by PubChem | |
Molecular Formula |
CaO | |
Source | PubChem | |
URL | https://pubchem.ncbi.nlm.nih.gov | |
Description | Data deposited in or computed by PubChem | |
DSSTOX Substance ID |
DTXSID5029631 | |
Record name | Calcium oxide | |
Source | EPA DSSTox | |
URL | https://comptox.epa.gov/dashboard/DTXSID5029631 | |
Description | DSSTox provides a high quality public chemistry resource for supporting improved predictive toxicology. | |
Molecular Weight |
56.08 g/mol | |
Source | PubChem | |
URL | https://pubchem.ncbi.nlm.nih.gov | |
Description | Data deposited in or computed by PubChem | |
Product Name |
Lime (Calcium Oxide) | |
CAS RN |
73018-51-6, 1305-78-8 | |
Record name | 1,6-Octadien-3-ol, 3,7-dimethyl-, acid-isomerized | |
Source | EPA Chemicals under the TSCA | |
URL | https://www.epa.gov/chemicals-under-tsca | |
Description | EPA Chemicals under the Toxic Substances Control Act (TSCA) collection contains information on chemicals and their regulations under TSCA, including non-confidential content from the TSCA Chemical Substance Inventory and Chemical Data Reporting. | |
Record name | Calcium oxide | |
Source | EPA DSSTox | |
URL | https://comptox.epa.gov/dashboard/DTXSID5029631 | |
Description | DSSTox provides a high quality public chemistry resource for supporting improved predictive toxicology. | |
Record name | 1,6-Octadien-3-ol, 3,7-dimethyl-, acid-isomerized | |
Source | European Chemicals Agency (ECHA) | |
URL | https://echa.europa.eu/substance-information/-/substanceinfo/100.070.184 | |
Description | The European Chemicals Agency (ECHA) is an agency of the European Union which is the driving force among regulatory authorities in implementing the EU's groundbreaking chemicals legislation for the benefit of human health and the environment as well as for innovation and competitiveness. | |
Explanation | Use of the information, documents and data from the ECHA website is subject to the terms and conditions of this Legal Notice, and subject to other binding limitations provided for under applicable law, the information, documents and data made available on the ECHA website may be reproduced, distributed and/or used, totally or in part, for non-commercial purposes provided that ECHA is acknowledged as the source: "Source: European Chemicals Agency, http://echa.europa.eu/". Such acknowledgement must be included in each copy of the material. ECHA permits and encourages organisations and individuals to create links to the ECHA website under the following cumulative conditions: Links can only be made to webpages that provide a link to the Legal Notice page. | |
Record name | LIME (CALCIUM OXIDE) | |
Source | FDA Global Substance Registration System (GSRS) | |
URL | https://gsrs.ncats.nih.gov/ginas/app/beta/substances/C7X2M0VVNH | |
Description | The FDA Global Substance Registration System (GSRS) enables the efficient and accurate exchange of information on what substances are in regulated products. Instead of relying on names, which vary across regulatory domains, countries, and regions, the GSRS knowledge base makes it possible for substances to be defined by standardized, scientific descriptions. | |
Explanation | Unless otherwise noted, the contents of the FDA website (www.fda.gov), both text and graphics, are not copyrighted. They are in the public domain and may be republished, reprinted and otherwise used freely by anyone without the need to obtain permission from FDA. Credit to the U.S. Food and Drug Administration as the source is appreciated but not required. | |
Synthesis routes and methods I
Procedure details
Synthesis routes and methods II
Procedure details
Synthesis routes and methods III
Procedure details
Synthesis routes and methods IV
Procedure details
試験管内研究製品の免責事項と情報
BenchChemで提示されるすべての記事および製品情報は、情報提供を目的としています。BenchChemで購入可能な製品は、生体外研究のために特別に設計されています。生体外研究は、ラテン語の "in glass" に由来し、生物体の外で行われる実験を指します。これらの製品は医薬品または薬として分類されておらず、FDAから任何の医療状態、病気、または疾患の予防、治療、または治癒のために承認されていません。これらの製品を人間または動物に体内に導入する形態は、法律により厳格に禁止されています。これらのガイドラインに従うことは、研究と実験において法的および倫理的な基準の遵守を確実にするために重要です。