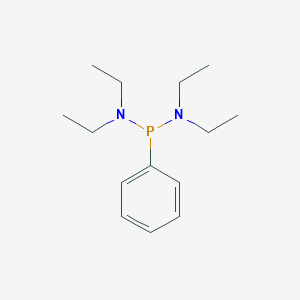
Bis(diethylamino)phenylphosphine
説明
Bis(diethylamino)phenylphosphine is a chemical compound with the molecular formula C14H25N2P . It is also known by other names such as N,N,N’N’-Tetraethylphenylphosphonous diamide and Phenylphosphonous tetraethyldiamide . It is used as a ligand in various chemical reactions .
Molecular Structure Analysis
The molecular weight of Bis(diethylamino)phenylphosphine is 252.34 g/mol . The SMILES string representation of its structure isCCN(CC)P(N(CC)CC)C1=CC=CC=C1
. Chemical Reactions Analysis
Bis(diethylamino)phenylphosphine is used as a ligand and is suitable for various types of coupling reactions, including Buchwald-Hartwig Cross Coupling, Heck Reaction, Hiyama Coupling, Negishi Coupling, Sonogashira Coupling, Stille Coupling, and Suzuki-Miyaura Coupling .Physical And Chemical Properties Analysis
Bis(diethylamino)phenylphosphine is a liquid with a density of 0.971 g/mL at 25 °C . Its boiling point is 80 °C at 0.005 mmHg .科学的研究の応用
Electron-Withdrawing Cocatalyst
“Bis(diethylamino)phenylphosphine” serves as an electron-withdrawing cocatalyst in rhodium-catalyzed hydroformylation reactions . Hydroformylation is a crucial process in the industrial production of aldehydes from alkenes. This compound enhances the efficiency of the reaction by withdrawing electron density from the metal center, thereby facilitating the oxidative addition step of the catalytic cycle .
Ligand Precatalyst for Heck Coupling Reactions
This compound acts as a ligand precatalyst for Heck coupling reactions . The Heck reaction is a palladium-catalyzed carbon-carbon cross-coupling reaction used in organic chemistry to synthesize substituted olefins . “Bis(diethylamino)phenylphosphine” enhances the efficiency of this reaction .
Preparation of Palladium Chiral P-N Ligand Complexes
“Bis(diethylamino)phenylphosphine” is used as a reactant for the preparation of palladium chiral P-N ligand complexes . These complexes are used for regio- and stereo-selective dimerization reactions . Dimerization reactions are important in the synthesis of complex organic compounds .
Preparation of Palladium Tautomeric Ferrocenylphosphinites
This compound is also used in the preparation of palladium tautomeric ferrocenylphosphinites . These are used as catalysts for Suzuki-Miyaura coupling . The Suzuki-Miyaura coupling is a type of palladium-catalyzed cross-coupling reaction, used to synthesize biaryl compounds .
Trimethylsilyl Halide-Promoted Michaelis-Arbuzov Rearrangement
“Bis(diethylamino)phenylphosphine” is used in the Trimethylsilyl halide-promoted Michaelis-Arbuzov rearrangement of phosphinites and phosphites . The Michaelis-Arbuzov reaction is an organic reaction that involves the rearrangement of a phosphite ester to a phosphonate ester .
Synthesis of Complex Organic Compounds
Due to its unique properties, “Bis(diethylamino)phenylphosphine” is used in the synthesis of complex organic compounds . Its ability to act as a ligand and a catalyst makes it a versatile tool in the field of organic synthesis .
作用機序
Target of Action
Bis(diethylamino)phenylphosphine, also known as N-[diethylamino(phenyl)phosphanyl]-N-ethylethanamine, is primarily used as a ligand and cocatalyst in various chemical reactions . It plays a crucial role in the catalysis of reactions such as the Buchwald-Hartwig Cross Coupling, Heck Reaction, Hiyama Coupling, Negishi Coupling, Sonogashira Coupling, Stille Coupling, and Suzuki-Miyaura Coupling .
Mode of Action
As a ligand, Bis(diethylamino)phenylphosphine binds to the active site of a catalyst, thereby facilitating the catalytic reaction . It acts as an electron-withdrawing cocatalyst in rhodium-catalyzed hydroformylation reactions . It also serves as a ligand precatalyst for Heck coupling reactions .
Biochemical Pathways
Bis(diethylamino)phenylphosphine is involved in the preparation of palladium chiral P-N ligand complexes for regio- and stereo-selective dimerization reactions . It also participates in the preparation of palladium tautomeric ferrocenylphosphinites as catalysts for Suzuki-Miyaura coupling . Additionally, it is a reactant in the Trimethylsilyl halide-promoted Michaelis-Arbuzov rearrangement of phosphinites and phosphites .
Result of Action
The result of Bis(diethylamino)phenylphosphine’s action is the facilitation of various chemical reactions, leading to the formation of desired products. For instance, it aids in the formation of palladium chiral P-N ligand complexes and palladium tautomeric ferrocenylphosphinites .
特性
IUPAC Name |
N-[diethylamino(phenyl)phosphanyl]-N-ethylethanamine | |
---|---|---|
Source | PubChem | |
URL | https://pubchem.ncbi.nlm.nih.gov | |
Description | Data deposited in or computed by PubChem | |
InChI |
InChI=1S/C14H25N2P/c1-5-15(6-2)17(16(7-3)8-4)14-12-10-9-11-13-14/h9-13H,5-8H2,1-4H3 | |
Source | PubChem | |
URL | https://pubchem.ncbi.nlm.nih.gov | |
Description | Data deposited in or computed by PubChem | |
InChI Key |
HTIVDCMRYKVNJC-UHFFFAOYSA-N | |
Source | PubChem | |
URL | https://pubchem.ncbi.nlm.nih.gov | |
Description | Data deposited in or computed by PubChem | |
Canonical SMILES |
CCN(CC)P(C1=CC=CC=C1)N(CC)CC | |
Source | PubChem | |
URL | https://pubchem.ncbi.nlm.nih.gov | |
Description | Data deposited in or computed by PubChem | |
Molecular Formula |
C14H25N2P | |
Source | PubChem | |
URL | https://pubchem.ncbi.nlm.nih.gov | |
Description | Data deposited in or computed by PubChem | |
DSSTOX Substance ID |
DTXSID00167622 | |
Record name | Phenylbis(diethylamino)phosphine | |
Source | EPA DSSTox | |
URL | https://comptox.epa.gov/dashboard/DTXSID00167622 | |
Description | DSSTox provides a high quality public chemistry resource for supporting improved predictive toxicology. | |
Molecular Weight |
252.34 g/mol | |
Source | PubChem | |
URL | https://pubchem.ncbi.nlm.nih.gov | |
Description | Data deposited in or computed by PubChem | |
Product Name |
Phenylbis(diethylamino)phosphine | |
CAS RN |
1636-14-2 | |
Record name | Phenylbis(diethylamino)phosphine | |
Source | ChemIDplus | |
URL | https://pubchem.ncbi.nlm.nih.gov/substance/?source=chemidplus&sourceid=0001636142 | |
Description | ChemIDplus is a free, web search system that provides access to the structure and nomenclature authority files used for the identification of chemical substances cited in National Library of Medicine (NLM) databases, including the TOXNET system. | |
Record name | NSC244337 | |
Source | DTP/NCI | |
URL | https://dtp.cancer.gov/dtpstandard/servlet/dwindex?searchtype=NSC&outputformat=html&searchlist=244337 | |
Description | The NCI Development Therapeutics Program (DTP) provides services and resources to the academic and private-sector research communities worldwide to facilitate the discovery and development of new cancer therapeutic agents. | |
Explanation | Unless otherwise indicated, all text within NCI products is free of copyright and may be reused without our permission. Credit the National Cancer Institute as the source. | |
Record name | Phenylbis(diethylamino)phosphine | |
Source | EPA DSSTox | |
URL | https://comptox.epa.gov/dashboard/DTXSID00167622 | |
Description | DSSTox provides a high quality public chemistry resource for supporting improved predictive toxicology. | |
Retrosynthesis Analysis
AI-Powered Synthesis Planning: Our tool employs the Template_relevance Pistachio, Template_relevance Bkms_metabolic, Template_relevance Pistachio_ringbreaker, Template_relevance Reaxys, Template_relevance Reaxys_biocatalysis model, leveraging a vast database of chemical reactions to predict feasible synthetic routes.
One-Step Synthesis Focus: Specifically designed for one-step synthesis, it provides concise and direct routes for your target compounds, streamlining the synthesis process.
Accurate Predictions: Utilizing the extensive PISTACHIO, BKMS_METABOLIC, PISTACHIO_RINGBREAKER, REAXYS, REAXYS_BIOCATALYSIS database, our tool offers high-accuracy predictions, reflecting the latest in chemical research and data.
Strategy Settings
Precursor scoring | Relevance Heuristic |
---|---|
Min. plausibility | 0.01 |
Model | Template_relevance |
Template Set | Pistachio/Bkms_metabolic/Pistachio_ringbreaker/Reaxys/Reaxys_biocatalysis |
Top-N result to add to graph | 6 |
Feasible Synthetic Routes
Q & A
Q1: What is the main application of Bis(diethylamino)phenylphosphine in synthetic chemistry?
A1: Bis(diethylamino)phenylphosphine serves as a versatile reagent for synthesizing heterocyclic compounds, particularly 1,3,5,2λ3-triazaphosphorines. It reacts with N-thioamido amidines under reflux in toluene, releasing two molecules of dialkylamine and forming the desired triazaphosphorine ring system []. This reactivity highlights its utility in constructing phosphorus-containing heterocycles.
Q2: Can you provide the structural characterization data for Bis(diethylamino)phenylphosphine?
A2:
Molecular Formula: C14H25N2P []* Molecular Weight: 252.34 g/mol []* Physical State: Colorless liquid []* Boiling Point: 113–116 °C (2 Torr) [] * Density: 0.971 g cm−3 (25 °C) []* Refractive Index:* 1.5340 (20 °C) []
Q3: How does Bis(diethylamino)phenylphosphine contribute to the synthesis of optically active compounds?
A3: Bis(diethylamino)phenylphosphine plays a crucial role in synthesizing optically active P-chiral dioxophenylphospholane-borane complexes. For example, it reacts with methyl 2,6-di-O-benzyl-beta-D-galactopyranoside to form a carbohydrate-derived phosphinite with high diastereoselectivity []. This example demonstrates the potential of this compound in asymmetric synthesis and chiral molecule development.
Q4: Are there any known reactions involving Bis(diethylamino)phenylphosphine and transition metal complexes?
A4: Yes, Bis(diethylamino)phenylphosphine can act as a ligand in transition metal complexes. Research shows its incorporation into cyclopentadienyl manganese carbonyl complexes, like CpMn(CO)2(PPh(NEt2)2). This complex undergoes interesting P–N bond cleavage reactions upon treatment with halogen acids or fluorinating agents, leading to the formation of various substituted phosphine complexes []. These reactions highlight the reactivity of this compound and its potential in organometallic chemistry.
試験管内研究製品の免責事項と情報
BenchChemで提示されるすべての記事および製品情報は、情報提供を目的としています。BenchChemで購入可能な製品は、生体外研究のために特別に設計されています。生体外研究は、ラテン語の "in glass" に由来し、生物体の外で行われる実験を指します。これらの製品は医薬品または薬として分類されておらず、FDAから任何の医療状態、病気、または疾患の予防、治療、または治癒のために承認されていません。これらの製品を人間または動物に体内に導入する形態は、法律により厳格に禁止されています。これらのガイドラインに従うことは、研究と実験において法的および倫理的な基準の遵守を確実にするために重要です。