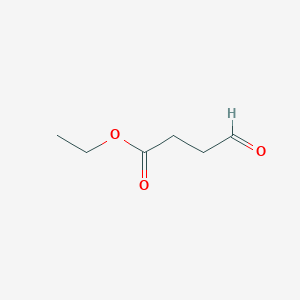
4-オキソブタン酸エチル
概要
説明
It is a versatile compound with the molecular formula C6H10O3 and a molecular weight of 130.14 g/mol . This compound is widely used in organic synthesis due to its reactivity and functional groups.
科学的研究の応用
Ethyl 4-oxobutanoate has numerous applications in scientific research:
作用機序
Target of Action
Ethyl 4-oxobutanoate, also known as ethyl 3-formylpropionate , is a carboxylic ester obtained by the formal condensation of the carboxy group of succinic semialdehyde with ethanol . It primarily targets enzymes such as carbonyl reductase (CgCR) and glucose dehydrogenase (GDH) . These enzymes play a crucial role in the biotransformation of ethyl 4-oxobutanoate into other compounds .
Mode of Action
The compound interacts with its targets through a process known as bioreduction . This process involves the highly stereoselective reduction of ethyl 4-oxobutanoate under mild conditions . The enolate ion of ethyl 4-oxobutanoate acts as a nucleophile and reacts with an electrophilic alkyl halide in an SN2 reaction, displacing the leaving group by backside attack .
Biochemical Pathways
The bioreduction of ethyl 4-oxobutanoate leads to the formation of optically active ®-ethyl 4-chloro-3-hydroxybutyrate (®-CHBE) . This compound is a useful chiral building block for the synthesis of pharmaceuticals . The process involves the transformation of ethyl 4-oxobutanoate into ®-CHBE via a highly efficient bioreduction process .
Result of Action
The result of the action of ethyl 4-oxobutanoate is the production of ®-CHBE . This compound is a key chiral precursor of enantiopure intermediates for synthesizing cholesterol-lowering hydroxymethylglutaryl-CoA reductase inhibitors .
Action Environment
The action of ethyl 4-oxobutanoate is influenced by environmental factors such as pH and temperature . For instance, at pH 7.0 and 30 °C, the kinetic constants Km and kcat of ethyl 4-oxobutanoate were found to be 20.9 mM and 56.1 s−1, respectively . Additionally, the presence of certain substances, such as Ni2+ and glucose, can enhance the bioreduction process .
準備方法
Synthetic Routes and Reaction Conditions: Ethyl 4-oxobutanoate can be synthesized through various methods. One common method involves the reaction of ethyl 4-pentenoate with ozone in dichloromethane at -78°C, followed by treatment with triphenylphosphine . Another method involves the alkylation of enolate ions, where ethyl acetoacetate is converted into its enolate ion by reaction with sodium ethoxide in ethanol, followed by alkylation with an alkyl halide .
Industrial Production Methods: In industrial settings, ethyl 4-oxobutanoate is typically produced through the esterification of succinic semialdehyde with ethanol. This process involves the use of acid catalysts to facilitate the reaction and achieve high yields .
化学反応の分析
Types of Reactions: Ethyl 4-oxobutanoate undergoes various chemical reactions, including:
Oxidation: It can be oxidized to form corresponding carboxylic acids.
Reduction: It can be reduced to form alcohols or aldehydes.
Substitution: It can undergo nucleophilic substitution reactions, particularly at the carbonyl carbon.
Common Reagents and Conditions:
Oxidation: Common oxidizing agents include potassium permanganate and chromium trioxide.
Reduction: Reducing agents such as sodium borohydride and lithium aluminum hydride are frequently used.
Substitution: Alkyl halides and strong bases like sodium hydride are commonly employed.
Major Products:
Oxidation: Succinic acid.
Reduction: 4-hydroxybutanoic acid or 4-hydroxybutanal.
Substitution: Various substituted esters depending on the alkyl halide used.
類似化合物との比較
Ethyl acetoacetate (ethyl 3-oxobutanoate): Similar in structure but has a different functional group arrangement.
Diethyl malonate: Another ester with similar reactivity but different applications.
Methyl 4-oxobutanoate: A methyl ester analog with similar chemical properties.
Uniqueness: Ethyl 4-oxobutanoate is unique due to its specific functional groups and reactivity, making it a valuable intermediate in organic synthesis. Its ability to undergo various chemical reactions and its applications in multiple fields highlight its versatility and importance in scientific research .
特性
IUPAC Name |
ethyl 4-oxobutanoate | |
---|---|---|
Source | PubChem | |
URL | https://pubchem.ncbi.nlm.nih.gov | |
Description | Data deposited in or computed by PubChem | |
InChI |
InChI=1S/C6H10O3/c1-2-9-6(8)4-3-5-7/h5H,2-4H2,1H3 | |
Source | PubChem | |
URL | https://pubchem.ncbi.nlm.nih.gov | |
Description | Data deposited in or computed by PubChem | |
InChI Key |
QFMPHCGACBODIJ-UHFFFAOYSA-N | |
Source | PubChem | |
URL | https://pubchem.ncbi.nlm.nih.gov | |
Description | Data deposited in or computed by PubChem | |
Canonical SMILES |
CCOC(=O)CCC=O | |
Source | PubChem | |
URL | https://pubchem.ncbi.nlm.nih.gov | |
Description | Data deposited in or computed by PubChem | |
Molecular Formula |
C6H10O3 | |
Source | PubChem | |
URL | https://pubchem.ncbi.nlm.nih.gov | |
Description | Data deposited in or computed by PubChem | |
DSSTOX Substance ID |
DTXSID90143879 | |
Record name | Ethyl 4-oxobutanoate | |
Source | EPA DSSTox | |
URL | https://comptox.epa.gov/dashboard/DTXSID90143879 | |
Description | DSSTox provides a high quality public chemistry resource for supporting improved predictive toxicology. | |
Molecular Weight |
130.14 g/mol | |
Source | PubChem | |
URL | https://pubchem.ncbi.nlm.nih.gov | |
Description | Data deposited in or computed by PubChem | |
CAS No. |
10138-10-0 | |
Record name | Butanoic acid, 4-oxo-, ethyl ester | |
Source | CAS Common Chemistry | |
URL | https://commonchemistry.cas.org/detail?cas_rn=10138-10-0 | |
Description | CAS Common Chemistry is an open community resource for accessing chemical information. Nearly 500,000 chemical substances from CAS REGISTRY cover areas of community interest, including common and frequently regulated chemicals, and those relevant to high school and undergraduate chemistry classes. This chemical information, curated by our expert scientists, is provided in alignment with our mission as a division of the American Chemical Society. | |
Explanation | The data from CAS Common Chemistry is provided under a CC-BY-NC 4.0 license, unless otherwise stated. | |
Record name | Ethyl 4-oxobutanoate | |
Source | ChemIDplus | |
URL | https://pubchem.ncbi.nlm.nih.gov/substance/?source=chemidplus&sourceid=0010138100 | |
Description | ChemIDplus is a free, web search system that provides access to the structure and nomenclature authority files used for the identification of chemical substances cited in National Library of Medicine (NLM) databases, including the TOXNET system. | |
Record name | Ethyl 4-oxobutanoate | |
Source | EPA DSSTox | |
URL | https://comptox.epa.gov/dashboard/DTXSID90143879 | |
Description | DSSTox provides a high quality public chemistry resource for supporting improved predictive toxicology. | |
Record name | ETHYL 4-OXOBUTANOATE | |
Source | FDA Global Substance Registration System (GSRS) | |
URL | https://gsrs.ncats.nih.gov/ginas/app/beta/substances/0592D2Y8Z0 | |
Description | The FDA Global Substance Registration System (GSRS) enables the efficient and accurate exchange of information on what substances are in regulated products. Instead of relying on names, which vary across regulatory domains, countries, and regions, the GSRS knowledge base makes it possible for substances to be defined by standardized, scientific descriptions. | |
Explanation | Unless otherwise noted, the contents of the FDA website (www.fda.gov), both text and graphics, are not copyrighted. They are in the public domain and may be republished, reprinted and otherwise used freely by anyone without the need to obtain permission from FDA. Credit to the U.S. Food and Drug Administration as the source is appreciated but not required. | |
Synthesis routes and methods I
Procedure details
Synthesis routes and methods II
Procedure details
Synthesis routes and methods III
Procedure details
Retrosynthesis Analysis
AI-Powered Synthesis Planning: Our tool employs the Template_relevance Pistachio, Template_relevance Bkms_metabolic, Template_relevance Pistachio_ringbreaker, Template_relevance Reaxys, Template_relevance Reaxys_biocatalysis model, leveraging a vast database of chemical reactions to predict feasible synthetic routes.
One-Step Synthesis Focus: Specifically designed for one-step synthesis, it provides concise and direct routes for your target compounds, streamlining the synthesis process.
Accurate Predictions: Utilizing the extensive PISTACHIO, BKMS_METABOLIC, PISTACHIO_RINGBREAKER, REAXYS, REAXYS_BIOCATALYSIS database, our tool offers high-accuracy predictions, reflecting the latest in chemical research and data.
Strategy Settings
Precursor scoring | Relevance Heuristic |
---|---|
Min. plausibility | 0.01 |
Model | Template_relevance |
Template Set | Pistachio/Bkms_metabolic/Pistachio_ringbreaker/Reaxys/Reaxys_biocatalysis |
Top-N result to add to graph | 6 |
Feasible Synthetic Routes
Q1: How does ethyl 4-oxobutanoate interact with Saccharomyces fermentati, and what are the downstream effects?
A1: While the exact mechanism of interaction isn't detailed in the provided research [, ], we know that Saccharomyces fermentati, a yeast species commonly used in fermentation processes, metabolizes ethyl 4-oxobutanoate when grown as a film on a simulated sherry medium []. This metabolic process results in the production of various compounds, including diethyl succinate, ethyl 4-hydroxybutanoate, and 4-hydroxybutanoic acid lactone. Additionally, the yeast produces 2-phenylethanol, 2- and 3-methylbutanol, and trace amounts of other unidentified compounds []. This metabolic transformation highlights the yeast's ability to modify and utilize ethyl 4-oxobutanoate as a substrate.
Q2: What is the structural characterization of ethyl 4-oxobutanoate?
A2: Although the provided research [, ] doesn't explicitly detail the spectroscopic data for ethyl 4-oxobutanoate, its molecular formula is C6H10O3, and its molecular weight is 130.14 g/mol. The compound is an ethyl ester with a ketone functional group on the butyrate chain.
Q3: Are there any analytical methods mentioned in the research used to study ethyl 4-oxobutanoate and its metabolites?
A3: Yes, the research highlights the use of gas chromatography coupled with various detection techniques to analyze ethyl 4-oxobutanoate and its metabolites []. Researchers used gas chromatography retention times to initially identify the compounds. Further structural confirmation was achieved using infrared spectroscopy (IR), nuclear magnetic resonance spectroscopy (NMR), and mass spectrometry (MS) []. These analytical techniques showcase a comprehensive approach to characterizing the metabolic products.
試験管内研究製品の免責事項と情報
BenchChemで提示されるすべての記事および製品情報は、情報提供を目的としています。BenchChemで購入可能な製品は、生体外研究のために特別に設計されています。生体外研究は、ラテン語の "in glass" に由来し、生物体の外で行われる実験を指します。これらの製品は医薬品または薬として分類されておらず、FDAから任何の医療状態、病気、または疾患の予防、治療、または治癒のために承認されていません。これらの製品を人間または動物に体内に導入する形態は、法律により厳格に禁止されています。これらのガイドラインに従うことは、研究と実験において法的および倫理的な基準の遵守を確実にするために重要です。