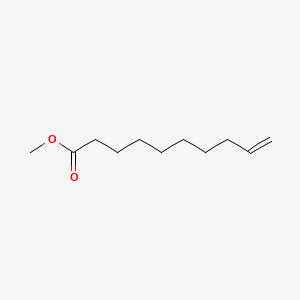
9-デセノ酸メチル
概要
説明
Methyl 9-decenoate is an organic compound with the molecular formula C11H20O2. It is an ester derived from 9-decenoic acid and methanol. This compound is characterized by a long carbon chain with a double bond at the ninth position and a methyl ester functional group. Methyl 9-decenoate is commonly used in various chemical reactions and industrial applications due to its unique structure and reactivity .
科学的研究の応用
Methyl 9-decenoate has a wide range of applications in scientific research:
Chemistry: It is used as a building block in the synthesis of various organic compounds, including polymers and pharmaceuticals.
Biology: Methyl 9-decenoate is studied for its potential biological activities, such as antimicrobial and anti-inflammatory properties.
Medicine: It is explored for its use in drug delivery systems and as a precursor for bioactive molecules.
Industry: Methyl 9-decenoate is used in the production of lubricants, plasticizers, and surfactants
作用機序
Target of Action
Methyl 9-decenoate is a chemical compound that primarily targets the combustion process in engines, particularly those that use biodiesel . This compound plays a crucial role in the combustion chemistry process of biodiesel, which is of great interest to engine researchers .
Mode of Action
The interaction of Methyl 9-decenoate with its targets involves a series of chemical reactions. During the combustion process, Methyl 9-decenoate undergoes various reactions such as dehydrogenation, oxygen addition, and molecular isomerization . These reactions result in changes in the molecular structure of Methyl 9-decenoate, contributing to the combustion process .
Biochemical Pathways
Methyl 9-decenoate affects the biochemical pathways involved in the combustion process. The low-temperature and high-temperature reaction pathways are particularly impacted . In the low-temperature stage, Methyl 9-decenoate is primarily consumed through dehydrogenation, oxygen addition, and molecular isomerization . In the high-temperature stage, the intermediate products from the low-temperature reactions undergo further reactions, such as C-O and C-C bond beta-decomposition, partial high-temperature dehydrogenation, and isomerization, to form small molecules like C2H4 .
Pharmacokinetics
While the term “pharmacokinetics” is typically used in the context of drug metabolism, in the case of Methyl 9-decenoate, we can discuss its behavior during the combustion processIts bioavailability can be inferred from its participation and consumption in the combustion reactions .
Result of Action
The molecular and cellular effects of Methyl 9-decenoate’s action are manifested in the changes it undergoes during the combustion process. The reactions involving Methyl 9-decenoate lead to the formation of smaller molecules, contributing to the energy output of the combustion process .
Action Environment
The action, efficacy, and stability of Methyl 9-decenoate are influenced by various environmental factors. These include the temperature and pressure conditions within the engine, which can affect the rate and efficiency of the combustion reactions involving Methyl 9-decenoate . For instance, the rate of the reactions involving Methyl 9-decenoate can increase with higher temperatures .
生化学分析
Biochemical Properties
Methyl 9-decenoate plays a crucial role in biochemical reactions, particularly in the context of biofuel production. It interacts with enzymes such as lipases, which catalyze the hydrolysis of ester bonds in lipids. This interaction is essential for the breakdown and utilization of fatty acid methyl esters in metabolic processes. Additionally, methyl 9-decenoate can undergo oxidation reactions mediated by cytochrome P450 enzymes, leading to the formation of epoxides and other oxidized derivatives .
Cellular Effects
Methyl 9-decenoate influences various cellular processes, including cell signaling pathways, gene expression, and cellular metabolism. It has been observed to modulate the activity of peroxisome proliferator-activated receptors (PPARs), which are nuclear receptors involved in the regulation of lipid metabolism and inflammation. By activating PPARs, methyl 9-decenoate can enhance the expression of genes involved in fatty acid oxidation and energy production . This compound also affects cellular signaling pathways by interacting with membrane-bound receptors and influencing downstream signaling cascades.
Molecular Mechanism
The molecular mechanism of action of methyl 9-decenoate involves its binding interactions with various biomolecules. It can act as a ligand for nuclear receptors such as PPARs, leading to changes in gene expression. Additionally, methyl 9-decenoate can inhibit or activate enzymes involved in lipid metabolism, such as lipases and cytochrome P450 enzymes. These interactions result in the modulation of metabolic pathways and the production of bioactive metabolites .
Temporal Effects in Laboratory Settings
In laboratory settings, the effects of methyl 9-decenoate can change over time due to its stability and degradation. Studies have shown that this compound is relatively stable under standard laboratory conditions, but it can undergo degradation when exposed to high temperatures or oxidative stress. Long-term exposure to methyl 9-decenoate has been associated with alterations in cellular function, including changes in lipid metabolism and energy production .
Dosage Effects in Animal Models
The effects of methyl 9-decenoate vary with different dosages in animal models. At low doses, it has been shown to enhance lipid metabolism and improve energy production. At high doses, methyl 9-decenoate can exhibit toxic effects, including liver damage and oxidative stress. Threshold effects have been observed, where the beneficial effects of methyl 9-decenoate are seen at lower doses, while adverse effects become prominent at higher doses .
準備方法
Synthetic Routes and Reaction Conditions: Methyl 9-decenoate is typically synthesized through olefin metathesis reactions. One common method involves the metathesis of unsaturated fatty acids, such as methyl oleate, using ruthenium-based catalysts. The reaction conditions often include mild temperatures and pressures to ensure high selectivity and yield .
Industrial Production Methods: In industrial settings, methyl 9-decenoate is produced using large-scale metathesis processes. These processes utilize renewable feedstocks like vegetable oils and fatty acid methyl esters. The metathesis reaction is followed by purification steps to isolate the desired product .
化学反応の分析
Types of Reactions: Methyl 9-decenoate undergoes various chemical reactions, including:
Oxidation: The double bond in methyl 9-decenoate can be oxidized to form epoxides or diols.
Reduction: The ester group can be reduced to form alcohols.
Substitution: The ester group can undergo nucleophilic substitution reactions to form different esters or amides.
Common Reagents and Conditions:
Oxidation: Reagents like peracids or hydrogen peroxide are used under mild conditions.
Reduction: Catalytic hydrogenation or metal hydrides are commonly employed.
Substitution: Nucleophiles such as amines or alcohols are used in the presence of catalysts.
Major Products Formed:
Epoxides and diols: from oxidation.
Alcohols: from reduction.
Amides and different esters: from substitution reactions.
類似化合物との比較
Methyl oleate: An ester of oleic acid with a similar structure but a longer carbon chain.
Methyl linoleate: An ester of linoleic acid with two double bonds.
Methyl stearate: A saturated ester with no double bonds.
Uniqueness: Methyl 9-decenoate is unique due to its specific position of the double bond and the presence of the ester group. This combination allows for selective reactions and makes it a valuable intermediate in organic synthesis. Compared to similar compounds, methyl 9-decenoate offers a balance of reactivity and stability, making it suitable for various applications .
特性
IUPAC Name |
methyl dec-9-enoate | |
---|---|---|
Source | PubChem | |
URL | https://pubchem.ncbi.nlm.nih.gov | |
Description | Data deposited in or computed by PubChem | |
InChI |
InChI=1S/C11H20O2/c1-3-4-5-6-7-8-9-10-11(12)13-2/h3H,1,4-10H2,2H3 | |
Source | PubChem | |
URL | https://pubchem.ncbi.nlm.nih.gov | |
Description | Data deposited in or computed by PubChem | |
InChI Key |
SBIGSHCJXYGFMX-UHFFFAOYSA-N | |
Source | PubChem | |
URL | https://pubchem.ncbi.nlm.nih.gov | |
Description | Data deposited in or computed by PubChem | |
Canonical SMILES |
COC(=O)CCCCCCCC=C | |
Source | PubChem | |
URL | https://pubchem.ncbi.nlm.nih.gov | |
Description | Data deposited in or computed by PubChem | |
Molecular Formula |
C11H20O2 | |
Source | PubChem | |
URL | https://pubchem.ncbi.nlm.nih.gov | |
Description | Data deposited in or computed by PubChem | |
DSSTOX Substance ID |
DTXSID30365139 | |
Record name | methyl 9-decenoate | |
Source | EPA DSSTox | |
URL | https://comptox.epa.gov/dashboard/DTXSID30365139 | |
Description | DSSTox provides a high quality public chemistry resource for supporting improved predictive toxicology. | |
Molecular Weight |
184.27 g/mol | |
Source | PubChem | |
URL | https://pubchem.ncbi.nlm.nih.gov | |
Description | Data deposited in or computed by PubChem | |
CAS No. |
25601-41-6 | |
Record name | Methyl 9-decenoate | |
Source | CAS Common Chemistry | |
URL | https://commonchemistry.cas.org/detail?cas_rn=25601-41-6 | |
Description | CAS Common Chemistry is an open community resource for accessing chemical information. Nearly 500,000 chemical substances from CAS REGISTRY cover areas of community interest, including common and frequently regulated chemicals, and those relevant to high school and undergraduate chemistry classes. This chemical information, curated by our expert scientists, is provided in alignment with our mission as a division of the American Chemical Society. | |
Explanation | The data from CAS Common Chemistry is provided under a CC-BY-NC 4.0 license, unless otherwise stated. | |
Record name | Methyl 9-decenoate | |
Source | ChemIDplus | |
URL | https://pubchem.ncbi.nlm.nih.gov/substance/?source=chemidplus&sourceid=0025601416 | |
Description | ChemIDplus is a free, web search system that provides access to the structure and nomenclature authority files used for the identification of chemical substances cited in National Library of Medicine (NLM) databases, including the TOXNET system. | |
Record name | 9-Decenoic acid, methyl ester | |
Source | EPA Chemicals under the TSCA | |
URL | https://www.epa.gov/chemicals-under-tsca | |
Description | EPA Chemicals under the Toxic Substances Control Act (TSCA) collection contains information on chemicals and their regulations under TSCA, including non-confidential content from the TSCA Chemical Substance Inventory and Chemical Data Reporting. | |
Record name | methyl 9-decenoate | |
Source | EPA DSSTox | |
URL | https://comptox.epa.gov/dashboard/DTXSID30365139 | |
Description | DSSTox provides a high quality public chemistry resource for supporting improved predictive toxicology. | |
Record name | Methyl dec-9-enoate | |
Source | European Chemicals Agency (ECHA) | |
URL | https://echa.europa.eu/substance-information/-/substanceinfo/100.189.140 | |
Description | The European Chemicals Agency (ECHA) is an agency of the European Union which is the driving force among regulatory authorities in implementing the EU's groundbreaking chemicals legislation for the benefit of human health and the environment as well as for innovation and competitiveness. | |
Explanation | Use of the information, documents and data from the ECHA website is subject to the terms and conditions of this Legal Notice, and subject to other binding limitations provided for under applicable law, the information, documents and data made available on the ECHA website may be reproduced, distributed and/or used, totally or in part, for non-commercial purposes provided that ECHA is acknowledged as the source: "Source: European Chemicals Agency, http://echa.europa.eu/". Such acknowledgement must be included in each copy of the material. ECHA permits and encourages organisations and individuals to create links to the ECHA website under the following cumulative conditions: Links can only be made to webpages that provide a link to the Legal Notice page. | |
Record name | Methyl 9-decenoate | |
Source | FDA Global Substance Registration System (GSRS) | |
URL | https://gsrs.ncats.nih.gov/ginas/app/beta/substances/4K2M7ZTD5T | |
Description | The FDA Global Substance Registration System (GSRS) enables the efficient and accurate exchange of information on what substances are in regulated products. Instead of relying on names, which vary across regulatory domains, countries, and regions, the GSRS knowledge base makes it possible for substances to be defined by standardized, scientific descriptions. | |
Explanation | Unless otherwise noted, the contents of the FDA website (www.fda.gov), both text and graphics, are not copyrighted. They are in the public domain and may be republished, reprinted and otherwise used freely by anyone without the need to obtain permission from FDA. Credit to the U.S. Food and Drug Administration as the source is appreciated but not required. | |
Synthesis routes and methods I
Procedure details
Synthesis routes and methods II
Procedure details
Synthesis routes and methods III
Procedure details
Synthesis routes and methods IV
Procedure details
Retrosynthesis Analysis
AI-Powered Synthesis Planning: Our tool employs the Template_relevance Pistachio, Template_relevance Bkms_metabolic, Template_relevance Pistachio_ringbreaker, Template_relevance Reaxys, Template_relevance Reaxys_biocatalysis model, leveraging a vast database of chemical reactions to predict feasible synthetic routes.
One-Step Synthesis Focus: Specifically designed for one-step synthesis, it provides concise and direct routes for your target compounds, streamlining the synthesis process.
Accurate Predictions: Utilizing the extensive PISTACHIO, BKMS_METABOLIC, PISTACHIO_RINGBREAKER, REAXYS, REAXYS_BIOCATALYSIS database, our tool offers high-accuracy predictions, reflecting the latest in chemical research and data.
Strategy Settings
Precursor scoring | Relevance Heuristic |
---|---|
Min. plausibility | 0.01 |
Model | Template_relevance |
Template Set | Pistachio/Bkms_metabolic/Pistachio_ringbreaker/Reaxys/Reaxys_biocatalysis |
Top-N result to add to graph | 6 |
Feasible Synthetic Routes
Q1: What is methyl 9-decenoate, and how is it produced?
A1: Methyl 9-decenoate is a fatty acid methyl ester primarily produced through the ethenolysis of methyl oleate, a common component of vegetable oils like palm oil [, , ]. This reaction involves breaking the carbon-carbon double bond in methyl oleate and adding ethylene across it, yielding methyl 9-decenoate and 1-decene [, , ]. The reaction is typically catalyzed by ruthenium-based Grubbs catalysts [, ] or molybdenum-based catalysts [, ].
Q2: Why is there interest in producing methyl 9-decenoate from renewable sources?
A2: Methyl 9-decenoate is considered a valuable platform chemical. Its production from renewable resources like vegetable oils offers a sustainable alternative to petroleum-based routes [, , ]. This aligns with growing efforts to reduce reliance on fossil fuels and develop environmentally friendly processes [, , ].
Q3: What are the potential applications of methyl 9-decenoate?
A3: Methyl 9-decenoate is primarily considered a valuable building block for synthesizing various products:
- Monomer for Polymers: It can be used as a monomer for producing polymers with potential applications in enhanced oil recovery (EOR) [].
- Fine Chemicals and Pharmaceuticals: It serves as an intermediate in the production of pheromones and other fine chemicals []. It's also explored as a potential building block for poly(amide-hydroxyurethane)s, which have applications in various industries [].
- Biodiesel Surrogate: Methyl 9-decenoate is studied as a component of biodiesel surrogate fuels, helping researchers understand the combustion characteristics of biodiesel and improve engine design [, , , , , , , ].
Q4: What is the molecular formula and molecular weight of methyl 9-decenoate?
A4: The molecular formula of methyl 9-decenoate is C11H20O2, and its molecular weight is 184.28 g/mol.
Q5: What spectroscopic techniques are used to characterize methyl 9-decenoate?
A5: Several spectroscopic methods help identify and confirm the structure of methyl 9-decenoate:
- Nuclear Magnetic Resonance (NMR) Spectroscopy: This technique provides detailed information about the carbon-hydrogen framework of the molecule [].
- Fourier Transform Infrared (FTIR) Spectroscopy: FTIR helps identify functional groups present in the molecule, such as the ester group (C=O) and the double bond (C=C) in methyl 9-decenoate [].
- Gas Chromatography-Mass Spectrometry (GC-MS): GC-MS is used to separate and identify methyl 9-decenoate in a mixture and confirm its molecular weight [].
Q6: How does the choice of catalyst influence the ethenolysis of methyl oleate to produce methyl 9-decenoate?
A6: Different catalysts exhibit varying activity, selectivity, and stability in the ethenolysis reaction.
- Molybdenum-Based Catalysts: Molybdenum-based catalysts, particularly MonoAryloxide-Pyrrolide (MAP) complexes, are also explored for their high Z-selectivity in olefin metathesis reactions, including ethenolysis [, ]. They have shown promising activity and selectivity in converting methyl oleate to methyl 9-decenoate [, ].
Q7: What factors can affect the yield and selectivity of methyl 9-decenoate in ethenolysis reactions?
A7: Several factors can significantly impact the reaction outcome:
- Temperature: The reaction temperature also plays a role. While higher temperatures can increase reaction rates, they can also negatively impact the equilibrium conversion of methyl oleate [].
Q8: How is computational chemistry used in research related to methyl 9-decenoate?
A8: Computational methods are valuable tools for understanding reaction mechanisms, predicting reaction outcomes, and designing new catalysts. For instance:
- Kinetic Modeling: Researchers use kinetic models to simulate the ethenolysis reaction, studying the influence of different parameters on reaction rates and product yields [].
- Molecular Modeling: Molecular modeling techniques help visualize and understand the interaction between catalysts and reactants at a molecular level, providing insights into the origin of selectivity in metathesis reactions [].
- Combustion Simulations: Computational fluid dynamics (CFD) simulations, coupled with chemical kinetics mechanisms for biodiesel surrogates like methyl 9-decenoate, are used to study the combustion characteristics of biodiesel fuels in engines [, , ]. These simulations help in predicting engine performance, emissions, and optimize engine designs for biodiesel utilization [, , ].
Q9: Are there any efforts to improve the sustainability of methyl 9-decenoate production?
A10: Research focuses on developing more efficient catalysts that operate under milder conditions, reducing energy consumption and waste generation []. Exploring alternative feedstocks for biodiesel production, such as waste cooking oils, can also contribute to the sustainability of the process [].
試験管内研究製品の免責事項と情報
BenchChemで提示されるすべての記事および製品情報は、情報提供を目的としています。BenchChemで購入可能な製品は、生体外研究のために特別に設計されています。生体外研究は、ラテン語の "in glass" に由来し、生物体の外で行われる実験を指します。これらの製品は医薬品または薬として分類されておらず、FDAから任何の医療状態、病気、または疾患の予防、治療、または治癒のために承認されていません。これらの製品を人間または動物に体内に導入する形態は、法律により厳格に禁止されています。これらのガイドラインに従うことは、研究と実験において法的および倫理的な基準の遵守を確実にするために重要です。