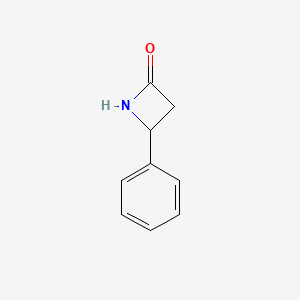
4-Phenylazetidin-2-one
概要
説明
4-Phenylazetidin-2-one is a chemical compound belonging to the class of azetidinones, which are four-membered lactams. This compound is characterized by the presence of a phenyl group attached to the azetidinone ring.
準備方法
Synthetic Routes and Reaction Conditions: The synthesis of 4-Phenylazetidin-2-one can be achieved through several methods. One common approach involves the Staudinger reaction, where hydrobenzamide reacts with acetoxyacetyl chloride in the presence of a base to form the intermediate β-lactam, which is then deprotected under reductive or hydrolytic conditions . Another method involves the iodine-catalyzed synthesis under microwave irradiation, which provides a rapid and efficient route to the compound .
Industrial Production Methods: Industrial production of this compound typically involves large-scale Staudinger reactions, optimized for high yield and purity. The reaction conditions are carefully controlled to ensure the efficient formation of the desired product, with overall yields reaching up to 80% .
化学反応の分析
Types of Reactions: 4-Phenylazetidin-2-one undergoes various chemical reactions, including:
Oxidation: The compound can be oxidized to form corresponding oxides.
Reduction: Reduction reactions can yield amines or other reduced forms.
Substitution: The azetidinone ring can undergo substitution reactions, particularly at the phenyl group or the nitrogen atom.
Common Reagents and Conditions:
Oxidation: Common oxidizing agents include potassium permanganate and chromium trioxide.
Reduction: Reducing agents such as lithium aluminum hydride or sodium borohydride are used.
Substitution: Substitution reactions often involve halogenating agents or nucleophiles under basic or acidic conditions.
Major Products: The major products formed from these reactions include various substituted azetidinones, amines, and oxides, depending on the specific reaction conditions and reagents used .
科学的研究の応用
Medicinal Chemistry
4-Phenylazetidin-2-one serves as a crucial intermediate in the synthesis of various pharmaceuticals. Its unique structural features allow it to participate in reactions that lead to biologically active compounds.
Synthesis of Bioactive Compounds
Research indicates that derivatives of this compound have been synthesized to enhance their biological activity. For instance, a series of 3-chloro-1-[4-(3-oxo-3H-benzo[f]chromen)-2-yl-1,3-thiazolyl]-4-phenylazetidin-2-one derivatives were developed and evaluated for antimicrobial and anti-inflammatory activities. The presence of specific substituents significantly influenced their efficacy against pathogens such as Klebsiella pneumoniae .
Anticancer Activity
Recent studies have demonstrated that certain derivatives exhibit promising anticancer properties. For example, a study involving various substituted phenylazetidin-2-one derivatives showed substantial cytotoxic effects against the MCF-7 breast cancer cell line, with inhibition percentages ranging from 89.84% to 94.76% . This suggests that modifications to the azetidinone structure can enhance its therapeutic potential.
Biological Studies
The biological applications of this compound extend beyond medicinal chemistry into areas such as microbiology and immunology.
Antimicrobial Properties
Various studies have documented the antimicrobial effects of this compound derivatives. For example, compounds synthesized from this core structure were tested for their ability to inhibit bacterial growth, showing significant activity against both Gram-positive and Gram-negative bacteria .
Immunostimulatory Effects
Some derivatives have been identified as immunostimulants, contributing to enhanced immune responses in vitro. This property makes them candidates for further exploration in vaccine development or immune-modulating therapies .
Industrial Applications
In addition to its pharmaceutical significance, this compound is utilized in various industrial applications.
Synthesis of Fine Chemicals
The compound is employed as a building block in organic synthesis, facilitating the production of complex molecules used in fine chemical manufacturing . Its versatility allows chemists to create a wide range of derivatives tailored for specific applications.
Material Science
Research has explored the use of this compound in developing new materials with unique properties, particularly in polymer chemistry where its incorporation can lead to enhanced material performance .
Case Studies
作用機序
The mechanism of action of 4-Phenylazetidin-2-one involves its interaction with specific molecular targets and pathways. For instance, in the context of its antimicrobial activity, the compound inhibits bacterial cell wall synthesis by targeting penicillin-binding proteins. This leads to the disruption of cell wall integrity and ultimately bacterial cell death . In anticancer applications, it may induce apoptosis through the stabilization of microtubules and inhibition of key signaling pathways .
類似化合物との比較
4-Phenylazetidin-2-one can be compared with other azetidinones and β-lactams:
生物活性
4-Phenylazetidin-2-one, a member of the azetidinone class, has garnered significant attention due to its diverse biological activities. This compound is characterized by a four-membered lactam ring, which is known for its role in various pharmacological applications, particularly in antimicrobial and anticancer therapies. This article reviews the biological activity of this compound, focusing on its antimicrobial properties, cytotoxic effects against cancer cell lines, and structure-activity relationships (SAR).
Antimicrobial Activity
Numerous studies have reported the antimicrobial potential of this compound derivatives against various bacterial and fungal strains. The compound exhibits significant activity due to its ability to inhibit the growth of both Gram-positive and Gram-negative bacteria.
Key Findings:
- Antibacterial Effects : Research indicates that this compound derivatives demonstrate potent antibacterial activity against strains such as Staphylococcus aureus and Escherichia coli. In one study, certain derivatives showed minimum inhibitory concentration (MIC) values comparable to standard antibiotics like ciprofloxacin .
- Antifungal Activity : The compound also exhibits antifungal properties, particularly against Candida albicans and Aspergillus niger. The antifungal activity was evaluated using similar methodologies as those for antibacterial testing, confirming its broad-spectrum efficacy .
- Comparison of Activity : A comparative analysis of various synthesized derivatives revealed that those with electron-withdrawing groups at the para position exhibited enhanced antimicrobial activity. For instance, compounds with p-chloro or p-nitro substitutions showed significant potency .
Table 1: Antimicrobial Activity of this compound Derivatives
Compound | Bacterial Strain | MIC (μg/mL) | Fungal Strain | MIC (μg/mL) |
---|---|---|---|---|
4e | S. aureus | 12.5 | A. niger | 25 |
4n | E. coli | 25 | C. albicans | 20 |
AZ-5 | Pseudomonas aeruginosa | 15 | - | - |
AZ-10 | Staphylococcus pyogenes | 10 | - | - |
Cytotoxicity Against Cancer Cell Lines
The anticancer potential of this compound has been explored through various studies focusing on its cytotoxic effects against different cancer cell lines.
Key Findings:
- Breast Cancer Cell Line (MCF-7) : Several derivatives showed high cytotoxicity against the MCF-7 breast cancer cell line, with inhibition percentages ranging from 89% to 94% at varying concentrations . The use of MTT assays confirmed these findings.
- Structure-Activity Relationships : The presence of specific substituents on the azetidinone ring significantly influences cytotoxic effects. For example, derivatives with electron-withdrawing groups at the para position demonstrated enhanced cytotoxicity compared to their counterparts .
Table 2: Cytotoxic Activity Against MCF-7 Cell Line
Compound | Concentration (μM) | % Inhibition |
---|---|---|
AZ-5 | 0.1 | 93.28 |
AZ-9 | 0.5 | 90.56 |
AZ-10 | 1 | 93.14 |
AZ-19 | 2 | 94.76 |
特性
IUPAC Name |
4-phenylazetidin-2-one | |
---|---|---|
Source | PubChem | |
URL | https://pubchem.ncbi.nlm.nih.gov | |
Description | Data deposited in or computed by PubChem | |
InChI |
InChI=1S/C9H9NO/c11-9-6-8(10-9)7-4-2-1-3-5-7/h1-5,8H,6H2,(H,10,11) | |
Source | PubChem | |
URL | https://pubchem.ncbi.nlm.nih.gov | |
Description | Data deposited in or computed by PubChem | |
InChI Key |
MWKMQPSNTJCASD-UHFFFAOYSA-N | |
Source | PubChem | |
URL | https://pubchem.ncbi.nlm.nih.gov | |
Description | Data deposited in or computed by PubChem | |
Canonical SMILES |
C1C(NC1=O)C2=CC=CC=C2 | |
Source | PubChem | |
URL | https://pubchem.ncbi.nlm.nih.gov | |
Description | Data deposited in or computed by PubChem | |
Molecular Formula |
C9H9NO | |
Source | PubChem | |
URL | https://pubchem.ncbi.nlm.nih.gov | |
Description | Data deposited in or computed by PubChem | |
DSSTOX Substance ID |
DTXSID40340957 | |
Record name | 4-phenylazetidin-2-one | |
Source | EPA DSSTox | |
URL | https://comptox.epa.gov/dashboard/DTXSID40340957 | |
Description | DSSTox provides a high quality public chemistry resource for supporting improved predictive toxicology. | |
Molecular Weight |
147.17 g/mol | |
Source | PubChem | |
URL | https://pubchem.ncbi.nlm.nih.gov | |
Description | Data deposited in or computed by PubChem | |
CAS No. |
5661-55-2 | |
Record name | 4-phenylazetidin-2-one | |
Source | EPA DSSTox | |
URL | https://comptox.epa.gov/dashboard/DTXSID40340957 | |
Description | DSSTox provides a high quality public chemistry resource for supporting improved predictive toxicology. | |
Record name | 4-phenylazetidin-2-one | |
Source | European Chemicals Agency (ECHA) | |
URL | https://echa.europa.eu/information-on-chemicals | |
Description | The European Chemicals Agency (ECHA) is an agency of the European Union which is the driving force among regulatory authorities in implementing the EU's groundbreaking chemicals legislation for the benefit of human health and the environment as well as for innovation and competitiveness. | |
Explanation | Use of the information, documents and data from the ECHA website is subject to the terms and conditions of this Legal Notice, and subject to other binding limitations provided for under applicable law, the information, documents and data made available on the ECHA website may be reproduced, distributed and/or used, totally or in part, for non-commercial purposes provided that ECHA is acknowledged as the source: "Source: European Chemicals Agency, http://echa.europa.eu/". Such acknowledgement must be included in each copy of the material. ECHA permits and encourages organisations and individuals to create links to the ECHA website under the following cumulative conditions: Links can only be made to webpages that provide a link to the Legal Notice page. | |
Synthesis routes and methods
Procedure details
Retrosynthesis Analysis
AI-Powered Synthesis Planning: Our tool employs the Template_relevance Pistachio, Template_relevance Bkms_metabolic, Template_relevance Pistachio_ringbreaker, Template_relevance Reaxys, Template_relevance Reaxys_biocatalysis model, leveraging a vast database of chemical reactions to predict feasible synthetic routes.
One-Step Synthesis Focus: Specifically designed for one-step synthesis, it provides concise and direct routes for your target compounds, streamlining the synthesis process.
Accurate Predictions: Utilizing the extensive PISTACHIO, BKMS_METABOLIC, PISTACHIO_RINGBREAKER, REAXYS, REAXYS_BIOCATALYSIS database, our tool offers high-accuracy predictions, reflecting the latest in chemical research and data.
Strategy Settings
Precursor scoring | Relevance Heuristic |
---|---|
Min. plausibility | 0.01 |
Model | Template_relevance |
Template Set | Pistachio/Bkms_metabolic/Pistachio_ringbreaker/Reaxys/Reaxys_biocatalysis |
Top-N result to add to graph | 6 |
Feasible Synthetic Routes
Q1: What is the molecular formula and weight of 4-Phenylazetidin-2-one?
A1: The molecular formula of this compound is C9H9NO, and its molecular weight is 147.17 g/mol.
Q2: How can spectroscopic techniques confirm the structure of this compound?
A2: Techniques like FTIR, 1H NMR, and mass spectrometry provide structural insights. For instance, FTIR can confirm the presence of the β-lactam ring through its characteristic carbonyl stretching frequency. [], []
Q3: What is a significant application of this compound in pharmaceutical synthesis?
A3: this compound serves as a key intermediate in the synthesis of the Taxol® side chain, a crucial component of the anticancer drug Taxol. []
Q4: Can this compound be used to synthesize medium-ring nitrogen-containing compounds?
A4: Yes, researchers have demonstrated the synthesis of 7-, 8-, and 9-membered 1,5-diazacyclic ketones from this compound derivatives through transamidative ring expansion in liquid ammonia. []
Q5: How does N-substitution on the this compound ring impact its reactivity in lipase-catalyzed ring-opening reactions?
A5: Studies have shown that the type of N-protecting group on this compound significantly influences its reactivity in lipase-catalyzed ring-opening reactions with alcohols. [], []
Q6: How is the stereochemistry of this compound relevant to its applications?
A6: The stereochemistry at the C3 and C4 positions is crucial, particularly in pharmaceutical synthesis. For example, the synthesis of Taxol requires specific stereoisomers of the side chain, which can be derived from chiral this compound derivatives. [], []
Q7: Can this compound derivatives act as catalysts in organic reactions?
A7: While not directly acting as catalysts, derivatives of this compound, particularly those incorporating metal complexes, have shown potential in asymmetric synthesis. For example, salen-copper(II) complexes derived from this compound have been used in the asymmetric synthesis of β-lactams. []
Q8: Can enzymes be used to synthesize chiral this compound derivatives?
A8: Yes, lipases like Candida antarctica lipase B have demonstrated highly enantioselective ring-opening alcoholysis of 4-arylazetidin-2-ones, providing a route to enantioenriched β-amino acid esters. []
Q9: How do substituents on the phenyl ring of this compound influence its biological activity?
A9: Research indicates that the presence and nature of substituents on the phenyl ring can significantly impact the biological activity of this compound derivatives. For instance, the introduction of a nitro group at the para position of the phenyl ring in 3-acetamido-4-phenylazetidin-2-one led to increased inhibition of Staphylococcus aureus β-lactamase. []
Q10: Can modifications to the β-lactam ring nitrogen affect the activity of this compound derivatives?
A10: Yes, N-substitution can significantly influence the reactivity and biological activity of this compound. For example, N-acylated derivatives showed better reactivity in lipase-catalyzed ring-opening reactions. []
Q11: What factors might affect the stability of this compound and its derivatives?
A11: Factors like pH, temperature, and the presence of nucleophiles can influence the stability of β-lactams. Specific studies on the stability of this compound under various conditions would be needed to provide a detailed assessment. []
Q12: Have computational methods been employed to study this compound?
A12: Yes, computational modeling has been used to understand the mechanism of lipase-catalyzed ring-opening reactions of this compound. This modeling provided insights into the enantioselectivity of the reaction. []
Q13: Is there information available regarding the toxicity of this compound?
A13: The provided research papers primarily focus on synthetic and mechanistic aspects. Dedicated toxicological studies would be needed to assess the safety profile of this compound and its derivatives.
Q14: What analytical techniques are commonly used to characterize and quantify this compound?
A14: Techniques like NMR spectroscopy, FTIR, mass spectrometry, and elemental analysis are routinely employed for structural characterization. Additionally, chiral HPLC methods are crucial for separating and analyzing enantiomers, particularly in the context of asymmetric synthesis and enzymatic resolutions. []
試験管内研究製品の免責事項と情報
BenchChemで提示されるすべての記事および製品情報は、情報提供を目的としています。BenchChemで購入可能な製品は、生体外研究のために特別に設計されています。生体外研究は、ラテン語の "in glass" に由来し、生物体の外で行われる実験を指します。これらの製品は医薬品または薬として分類されておらず、FDAから任何の医療状態、病気、または疾患の予防、治療、または治癒のために承認されていません。これらの製品を人間または動物に体内に導入する形態は、法律により厳格に禁止されています。これらのガイドラインに従うことは、研究と実験において法的および倫理的な基準の遵守を確実にするために重要です。