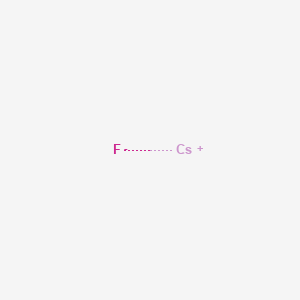
Cesium fluoride
説明
Cesium fluoride (CsF) is an inorganic compound with the formula CsF, characterized as a hygroscopic white crystalline solid. It has a molecular weight of 151.90 g/mol, a melting point of 682°C, and a boiling point of 1251°C . Its ionic nature arises from the large electronegativity difference between cesium (electronegativity: 0.79) and fluorine (3.98), resulting in a highly polar Cs–F bond . Structurally, CsF forms a cubic crystal lattice in its pure form, with Cs–F bond distances averaging ~3.01 Å in the solid state .
CsF is notable for its exceptional solubility in polar solvents (e.g., 367 g/100 mL in water at 25°C), surpassing other alkali metal fluorides like NaF and KF due to cesium’s low charge density and weak lattice energy . This property makes it a preferred fluoride source in organic synthesis, where it facilitates nucleophilic fluorination, silyl ether activations, and participation in SuFEx (Sulfur(VI) Fluoride Exchange) click chemistry . Additionally, CsF forms unique ammoniates, such as Cs₃F₃(NH₃)₄, which feature three-dimensional frameworks stabilized by N–H···F hydrogen bonds—a rarity among alkali metal halides .
準備方法
Traditional Synthesis Routes
Neutralization of Cesium Hydroxide with Hydrofluoric Acid
The classical method involves reacting aqueous cesium hydroxide (CsOH) with hydrofluoric acid (HF):
{(aq)} + \text{HF}{(aq)} \rightarrow \text{CsF}{(aq)} + \text{H}2\text{O}_{(l)}
This exothermic reaction proceeds at ambient temperatures, yielding CsF solutions that require evaporation and crystallization. While straightforward, the method suffers from inefficiencies: prolonged drying at 230–250°C is necessary to remove residual water, leading to high energy costs and potential decomposition of CsF into cesium oxyfluorides . Industrial implementations report product purities of 95–98%, with impurities primarily from incomplete neutralization and equipment corrosion .
Metathesis Reactions with Fluorinated Salts
Alternative approaches employ cesium carbonate (CsCO) or cesium sulfate (CsSO) as starting materials. For example:
2\text{CO}{3(s)} + 2\text{NH}4\text{F}{(aq)} \rightarrow 2\text{CsF}{(s)} + 2\text{NH}{3(g)} + \text{CO}{2(g)} + \text{H}2\text{O}_{(l)}
Ammonium fluoride (NHF) acts as the fluorine source, releasing gaseous byproducts that complicate reactor design. This method achieves higher yields (≥99%) but necessitates stringent control over stoichiometry and gas handling systems .
Advanced Solvent-Mediated Synthesis
Organic Solvent Systems for Enhanced Crystallization
A patented innovation (CN105540621A) circumvents aqueous-phase limitations by utilizing anhydrous ethanol or ethers as reaction media . Hydrogen fluoride gas (HF) is dissolved in the solvent at 10–50% w/w, followed by gradual addition of CsCO at 95–110% of stoichiometric requirements. Key advantages include:
-
Low-Temperature Processing : Reactions proceed at 25–40°C, avoiding thermal degradation.
-
Rapid Crystallization : CsF’s low solubility in organic solvents enables immediate precipitation, reducing energy-intensive concentration steps.
-
Solvent Recycling : Distillation at 80–120°C under vacuum (-0.01 to -0.08 MPa) recovers >90% of the organic phase, slashing raw material costs .
Table 1: Performance Comparison of CsF Synthesis Methods
pH Optimization and Impurity Control
The organic solvent method mandates precise pH regulation (3–7) during CsCO addition. Excess CsOH adjusts the final pH to 6.8–7.0, minimizing polyfluoro species like CsF or CsF that compromise purity . Centrifugation at 1,100 rpm and vacuum drying at 100°C yield anhydrous CsF with ≤0.1% residual solvent, meeting pharmaceutical-grade specifications .
Solid-State Synthesis and Vapor Deposition
High-Temperature Fluorination of Cesium Chloride
In aerospace applications, CsF thin films are deposited via chemical vapor deposition (CVD) using CsCl and SF:
{(g)} + \text{SF}{6(g)} \rightarrow \text{CsF}{(s)} + \text{SCl}{(g)} + \text{ClF}_{5(g)}
Operating at 600–800°C, this method produces ultra-pure CsF coatings for optical components. However, toxic byproducts (ClF) necessitate advanced scrubbing systems, limiting scalability .
Mechanochemical Synthesis
Ball milling CsOH with polytetrafluoroethylene (PTFE) at 300–400 rpm induces solid-state fluorination:
{(s)} + (\text{C}2\text{F}4)n \rightarrow \text{CsF}{(s)} + \text{CO}{(g)} + \text{H}2\text{O}{(g)}
This solvent-free approach achieves 85–90% conversion in 2–4 hours but requires post-milling thermal treatment to remove carbonaceous residues .
化学反応の分析
Types of Reactions: Cesium fluoride primarily acts as a source of fluoride ions in various chemical reactions. It undergoes several types of reactions, including:
Substitution Reactions: this compound is used in nucleophilic substitution reactions, where it replaces other halides with fluoride ions.
Desilylation Reactions: It is used to remove silyl protecting groups in organic synthesis.
Cross-Coupling Reactions: this compound is employed in cross-coupling reactions such as the Suzuki reaction.
Common Reagents and Conditions:
Nucleophilic Substitution: this compound reacts with electron-deficient aryl chlorides to form aryl fluorides.
Major Products Formed:
Aryl Fluorides: Formed from the reaction with aryl chlorides.
Organosilicon Fluorides: Produced during desilylation reactions.
科学的研究の応用
Catalysis
Cesium fluoride is recognized as a potent catalyst in organic synthesis. Its ability to act as a strong base makes it suitable for several key reactions:
- Organic Synthesis : CsF nanoparticles serve as basic catalysts in nucleophilic substitution reactions, facilitating the synthesis of organic fluorine compounds. This is particularly valuable in pharmaceutical and agrochemical manufacturing, where fluorinated compounds are often desired for their unique properties .
- Fluorination Reactions : CsF is effective in introducing fluorine into organic molecules, a critical step in the synthesis of various pharmaceuticals. The compound's reactivity at the nanoscale enhances its catalytic efficiency .
- Esterification Processes : Research indicates that this compound can promote the esterification of carboxylic acids, providing a practical alternative to traditional methods such as diazomethane reactions .
Photonics and Optical Applications
The optical properties of this compound make it a valuable material in photonics:
- Fluorescent Probes : CsF nanoparticles are being explored as fluorescent probes for bioimaging applications. Their ability to emit light makes them suitable for tracking cellular processes in real-time .
- Optical Devices : The compound is utilized in the development of light-emitting diodes (LEDs), lasers, and sensors. Its effectiveness in the ultraviolet spectrum enhances its application potential in various optical technologies .
Biomedical Applications
This compound nanoparticles have significant implications in medicine:
- Drug Delivery Systems : Due to their small size and high surface area, this compound nanoparticles can be engineered for targeted drug delivery, particularly in cancer therapy. Their surface can be modified to improve drug loading and release profiles .
- Biosensors : CsF nanoparticles are being investigated for their ability to detect specific biomolecules or pathogens. This capability is crucial for diagnostics and monitoring therapeutic drug levels .
Environmental Science
The application of this compound extends into environmental science:
- CO₂ Sequestration : Research has shown that this compound nanoparticles can react with carbon dioxide, making them candidates for carbon capture technologies. This application is vital for mitigating greenhouse gas emissions and addressing climate change challenges .
Case Studies and Research Findings
Several studies highlight the practical applications of this compound:
- A study on the catalytic properties of this compound demonstrated its effectiveness in enhancing reaction yields during organic synthesis, particularly when used as a promoter in esterification reactions .
- Research into the use of this compound nanoparticles for CO₂ capture revealed promising results, indicating their potential role in developing sustainable carbon management technologies .
作用機序
Cesium fluoride acts primarily as a source of fluoride ions. In organic synthesis, it facilitates reactions by providing fluoride ions that can attack electrophilic centers. For example, in desilylation reactions, this compound reacts with organosilicon compounds to produce organosilicon fluorides and carbanions, which can then react with electrophiles .
類似化合物との比較
Alkali Metal Fluorides: Structural and Physical Properties
The alkali metal fluorides (LiF, NaF, KF, RbF, CsF) share ionic bonding but exhibit distinct physical and chemical behaviors due to variations in cation size and polarizability.
Table 1: Comparative Properties of Alkali Metal Fluorides
Property | CsF | NaF | KF | RbF |
---|---|---|---|---|
Molecular Weight (g/mol) | 151.90 | 41.99 | 58.10 | 104.47 |
Melting Point (°C) | 682 | 993 | 858 | 795 |
Boiling Point (°C) | 1251 | 1704 | 1505 | 1410 |
Solubility in H₂O (g/100 mL, 25°C) | 367 | 4.2 | 92 | 130 |
Ionic Radius (Å) | Cs⁺: 1.88 | Na⁺: 1.16 | K⁺: 1.52 | Rb⁺: 1.66 |
Cs–F Bond Distance (Å) | 3.01–3.12 | Na–F: 2.31 | K–F: 2.67 | Rb–F: 2.82 |
Key Observations :
- Solubility : CsF’s solubility is ~87 times greater than NaF and ~4 times greater than KF due to weaker ionic interactions in its lattice .
- Thermal Stability : NaF has the highest melting point (993°C) owing to strong Coulombic forces between small Na⁺ and F⁻ ions. In contrast, CsF’s lower melting point (682°C) reflects weaker lattice energy .
- Coordination Chemistry : Cs⁺’s large ionic radius allows it to accommodate more ligands. For example, in ammoniates, CsF forms octahedral coordination with fluoride ions and ammonia molecules, a feature absent in NaF and KF .
Fluoride Ion Availability
CsF’s high solubility and low lattice energy enhance fluoride ion (F⁻) availability in solution, making it superior to NaF and KF in reactions requiring “naked” F⁻. For example, CsF catalyzes the synthesis of polysulfates via SuFEx chemistry by activating silyl ethers, whereas NaF and KF are ineffective under similar conditions .
Catalytic Versatility
- Organic Synthesis : CsF is widely used in nucleophilic fluorination, deprotection of silyl ethers, and cycloaddition reactions. In contrast, NaF is less reactive due to poor solubility, and KF often requires crown ethers to enhance F⁻ availability .
- Polymer Chemistry : CsF, in combination with tert-butoxide, enables controlled polymerization of fluorosulfates, whereas NaF and KF fail to initiate such reactions .
Solid-State Coordination
CsF participates in unique solid-state complexes. For instance, in polymeric copper(I) fluoride complexes, cesium ions occupy lattice cavities between copper-fluoride layers, stabilizing the structure through electrostatic interactions.
Toxicity and Environmental Impact
While fluoride toxicity is well-documented (e.g., reproductive toxicity via oxidative stress and hormonal disruption ), CsF’s environmental impact is less studied compared to NaF and KF. NaF is commonly linked to groundwater fluoride contamination, particularly in alkaline conditions where F⁻ solubility increases . NaF in water fluoridation) reduces ecological exposure .
生物活性
Cesium fluoride (CsF) is an inorganic compound that has garnered attention for its various applications in chemical synthesis and potential biological effects. This article delves into the biological activity of CsF, highlighting its mechanisms of action, effects on cellular systems, and implications for research and industry.
Overview of this compound
This compound is a highly soluble salt that dissociates in solution to release cesium ions (Cs⁺) and fluoride ions (F⁻). Its unique properties make it useful in various chemical reactions, particularly in organic synthesis as a fluoride source. Additionally, CsF has been studied for its potential biological interactions, particularly in the context of drug development and material science.
Mechanisms of Biological Activity
- Fluoride Ion Effects : The fluoride ion is known to influence various biological processes. It can inhibit certain enzymes, affect ion channels, and alter cellular signaling pathways. The specific impact of fluoride ions on biological systems is complex and can vary depending on concentration and exposure duration.
- Cesium Ion Effects : Cesium ions can mimic potassium ions in biological systems due to their similar size and charge. This can lead to disruptions in normal cellular functions, particularly in neuronal signaling and muscle contraction.
Enzymatic Inhibition
Research indicates that CsF can inhibit specific enzymes involved in metabolic pathways. For instance, studies have shown that fluoride ions can inhibit enolase, an enzyme critical for glycolysis, which may affect energy metabolism in cells .
Cellular Studies
A study investigated the effects of CsF on cell viability and proliferation in various cell lines. Results indicated that low concentrations of CsF could enhance cell proliferation due to its role as a fluoride source in synthetic pathways, while higher concentrations led to cytotoxic effects .
Case Studies
- Fluoride-Mediated Reactions : A study highlighted the use of CsF in facilitating N-methylation reactions in the presence of various substrates. The results showed that CsF could mediate significant structural changes in compounds, indicating its potential utility in drug development .
- Toxicological Assessments : Toxicological studies have demonstrated that exposure to high levels of CsF can lead to adverse effects such as neurotoxicity and disruption of calcium homeostasis in neurons. These findings underscore the importance of understanding the dosage and exposure levels when considering CsF for therapeutic applications .
Table 1: Summary of Biological Effects of this compound
Table 2: Applications of this compound in Research
Q & A
Q. Basic: What are the critical physicochemical properties of cesium fluoride (CsF) that influence its reactivity in synthetic chemistry?
Answer:
CsF exhibits high ionic character (due to the large electronegativity difference between Cs⁺ and F⁻), a cubic crystal structure, and exceptional solubility in polar solvents like water (~367 g/100 mL at 25°C). Its low lattice energy (~740 kJ/mol) enhances its role as a fluoride source in nucleophilic substitutions, while its hygroscopicity necessitates anhydrous handling in moisture-sensitive reactions . Reactivity is further modulated by its ability to stabilize intermediates via electrostatic interactions in organic transformations, such as aldol condensations .
Q. Basic: How can researchers ensure reproducibility in CsF-mediated reactions?
Answer:
Reproducibility requires strict control of:
- Moisture levels : Store CsF at 0–6°C in desiccators and pre-dry solvents (e.g., molecular sieves for THF) to prevent hydrolysis .
- Stoichiometry : Use precise gravimetric measurements (not volumetric) due to CsF’s hygroscopic nature.
- Reaction monitoring : Employ techniques like in situ IR spectroscopy to track fluoride release kinetics, which correlates with reaction progress .
Q. Advanced: How can contradictions in CsF’s catalytic efficiency across studies be resolved?
Answer:
Contradictions often arise from:
- Solvent effects : CsF’s solubility varies dramatically with solvent polarity (e.g., low in DMF vs. high in water), altering active fluoride availability. Compare studies using identical solvent systems .
- Impurity profiles : Trace hydroxide (OH⁻) in CsF can deactivate fluoride nucleophiles. Validate purity via X-ray diffraction (XRD) or ion chromatography .
- Substrate specificity : Steric hindrance in bulky substrates may limit CsF’s efficacy. Conduct kinetic isotope effect (KIE) studies to distinguish mechanistic pathways .
Q. Advanced: What computational models predict CsF’s behavior in solvent extraction systems?
Answer:
Extended equilibrium models, such as those for caustic-side solvent extraction (CSSX), integrate:
- Ion-pairing constants : Derived from UV-Vis titration data to quantify Cs⁺-F⁻ interactions in mixed-anion media (e.g., nitrate/fluoride systems) .
- Activity coefficients : Use Pitzer equations to account for high ionic strengths (>3 M) in waste simulants, which affect CsF’s partitioning .
- Machine learning : Train models on datasets spanning pH 2–12 and [Cs⁺] 0.1–1.5 M to predict extraction efficiency under untested conditions .
Q. Basic: What safety protocols are critical when handling CsF in laboratory settings?
Answer:
- Personal protective equipment (PPE) : Wear nitrile gloves, goggles, and lab coats to prevent dermal/ocular exposure. CsF’s corrosivity necessitates emergency eyewash stations .
- Ventilation : Use fume hoods for dry-powder handling to avoid inhalation of particulate matter.
- Waste disposal : Neutralize CsF solutions with calcium hydroxide (Ca(OH)₂) to precipitate less toxic CaF₂ before disposal .
Q. Advanced: How can spectroscopic techniques elucidate CsF’s structural dynamics in molten salt systems?
Answer:
- Raman spectroscopy : Identify Cs–F vibrational modes (200–400 cm⁻¹) to assess ion pairing vs. free ion dominance at temperatures >500°C .
- Neutron diffraction : Resolve coordination numbers and bond lengths in CsF-LiF eutectic mixtures, critical for nuclear reactor coolant designs .
- X-ray absorption near-edge structure (XANES) : Probe fluoride’s electronic environment in CsF-doped glasses for optoelectronic applications .
Q. Basic: What methodologies validate CsF’s purity and stoichiometry in synthesized samples?
Answer:
- Elemental analysis : Combustion analysis for fluorine content (theoretical F: 12.5%) with ±0.3% error .
- Thermogravimetric analysis (TGA) : Detect moisture or hydroxide impurities via mass loss profiles below 200°C .
- ICP-OES : Quantify cesium (Cs⁺) concentrations against certified reference materials (e.g., NIST SRM 3128) .
Q. Advanced: What strategies optimize CsF’s role in fluoride-ion batteries (FIBs)?
Answer:
- Electrolyte design : Blend CsF with ionic liquids (e.g., [EMIM][BF₄]) to enhance ionic conductivity (>10 mS/cm at 60°C) while suppressing dendrite formation .
- Cathode engineering : Pair CsF with transition metal fluorides (e.g., FeF₃) via ball-milling to create nanocomposites with improved cycling stability (>500 cycles) .
- Operando XRD : Monitor phase transitions during charge/discharge to identify degradation mechanisms (e.g., CsF agglomeration) .
特性
IUPAC Name |
cesium;fluoride | |
---|---|---|
Source | PubChem | |
URL | https://pubchem.ncbi.nlm.nih.gov | |
Description | Data deposited in or computed by PubChem | |
InChI |
InChI=1S/Cs.FH/h;1H/q+1;/p-1 | |
Source | PubChem | |
URL | https://pubchem.ncbi.nlm.nih.gov | |
Description | Data deposited in or computed by PubChem | |
InChI Key |
XJHCXCQVJFPJIK-UHFFFAOYSA-M | |
Source | PubChem | |
URL | https://pubchem.ncbi.nlm.nih.gov | |
Description | Data deposited in or computed by PubChem | |
Canonical SMILES |
[F-].[Cs+] | |
Source | PubChem | |
URL | https://pubchem.ncbi.nlm.nih.gov | |
Description | Data deposited in or computed by PubChem | |
Molecular Formula |
CsF | |
Record name | caesium fluoride | |
Source | Wikipedia | |
URL | https://en.wikipedia.org/wiki/Caesium_fluoride | |
Description | Chemical information link to Wikipedia. | |
Source | PubChem | |
URL | https://pubchem.ncbi.nlm.nih.gov | |
Description | Data deposited in or computed by PubChem | |
DSSTOX Substance ID |
DTXSID30893927 | |
Record name | Cesium fluoride | |
Source | EPA DSSTox | |
URL | https://comptox.epa.gov/dashboard/DTXSID30893927 | |
Description | DSSTox provides a high quality public chemistry resource for supporting improved predictive toxicology. | |
Molecular Weight |
151.9038551 g/mol | |
Source | PubChem | |
URL | https://pubchem.ncbi.nlm.nih.gov | |
Description | Data deposited in or computed by PubChem | |
Physical Description |
Deliquescent solid; [Hawley] White odorless crystalline solid; [MSDSonline] | |
Record name | Cesium fluoride | |
Source | Haz-Map, Information on Hazardous Chemicals and Occupational Diseases | |
URL | https://haz-map.com/Agents/9099 | |
Description | Haz-Map® is an occupational health database designed for health and safety professionals and for consumers seeking information about the adverse effects of workplace exposures to chemical and biological agents. | |
Explanation | Copyright (c) 2022 Haz-Map(R). All rights reserved. Unless otherwise indicated, all materials from Haz-Map are copyrighted by Haz-Map(R). No part of these materials, either text or image may be used for any purpose other than for personal use. Therefore, reproduction, modification, storage in a retrieval system or retransmission, in any form or by any means, electronic, mechanical or otherwise, for reasons other than personal use, is strictly prohibited without prior written permission. | |
CAS No. |
13400-13-0 | |
Record name | Cesium fluoride | |
Source | CAS Common Chemistry | |
URL | https://commonchemistry.cas.org/detail?cas_rn=13400-13-0 | |
Description | CAS Common Chemistry is an open community resource for accessing chemical information. Nearly 500,000 chemical substances from CAS REGISTRY cover areas of community interest, including common and frequently regulated chemicals, and those relevant to high school and undergraduate chemistry classes. This chemical information, curated by our expert scientists, is provided in alignment with our mission as a division of the American Chemical Society. | |
Explanation | The data from CAS Common Chemistry is provided under a CC-BY-NC 4.0 license, unless otherwise stated. | |
Record name | Cesium fluoride | |
Source | ChemIDplus | |
URL | https://pubchem.ncbi.nlm.nih.gov/substance/?source=chemidplus&sourceid=0013400130 | |
Description | ChemIDplus is a free, web search system that provides access to the structure and nomenclature authority files used for the identification of chemical substances cited in National Library of Medicine (NLM) databases, including the TOXNET system. | |
Record name | CESIUM FLUORIDE | |
Source | DTP/NCI | |
URL | https://dtp.cancer.gov/dtpstandard/servlet/dwindex?searchtype=NSC&outputformat=html&searchlist=84270 | |
Description | The NCI Development Therapeutics Program (DTP) provides services and resources to the academic and private-sector research communities worldwide to facilitate the discovery and development of new cancer therapeutic agents. | |
Explanation | Unless otherwise indicated, all text within NCI products is free of copyright and may be reused without our permission. Credit the National Cancer Institute as the source. | |
Record name | Cesium fluoride (CsF) | |
Source | EPA Chemicals under the TSCA | |
URL | https://www.epa.gov/chemicals-under-tsca | |
Description | EPA Chemicals under the Toxic Substances Control Act (TSCA) collection contains information on chemicals and their regulations under TSCA, including non-confidential content from the TSCA Chemical Substance Inventory and Chemical Data Reporting. | |
Record name | Cesium fluoride | |
Source | EPA DSSTox | |
URL | https://comptox.epa.gov/dashboard/DTXSID30893927 | |
Description | DSSTox provides a high quality public chemistry resource for supporting improved predictive toxicology. | |
Record name | Caesium fluoride | |
Source | European Chemicals Agency (ECHA) | |
URL | https://echa.europa.eu/substance-information/-/substanceinfo/100.033.156 | |
Description | The European Chemicals Agency (ECHA) is an agency of the European Union which is the driving force among regulatory authorities in implementing the EU's groundbreaking chemicals legislation for the benefit of human health and the environment as well as for innovation and competitiveness. | |
Explanation | Use of the information, documents and data from the ECHA website is subject to the terms and conditions of this Legal Notice, and subject to other binding limitations provided for under applicable law, the information, documents and data made available on the ECHA website may be reproduced, distributed and/or used, totally or in part, for non-commercial purposes provided that ECHA is acknowledged as the source: "Source: European Chemicals Agency, http://echa.europa.eu/". Such acknowledgement must be included in each copy of the material. ECHA permits and encourages organisations and individuals to create links to the ECHA website under the following cumulative conditions: Links can only be made to webpages that provide a link to the Legal Notice page. | |
Record name | CESIUM FLUORIDE | |
Source | FDA Global Substance Registration System (GSRS) | |
URL | https://gsrs.ncats.nih.gov/ginas/app/beta/substances/T76A371HJR | |
Description | The FDA Global Substance Registration System (GSRS) enables the efficient and accurate exchange of information on what substances are in regulated products. Instead of relying on names, which vary across regulatory domains, countries, and regions, the GSRS knowledge base makes it possible for substances to be defined by standardized, scientific descriptions. | |
Explanation | Unless otherwise noted, the contents of the FDA website (www.fda.gov), both text and graphics, are not copyrighted. They are in the public domain and may be republished, reprinted and otherwise used freely by anyone without the need to obtain permission from FDA. Credit to the U.S. Food and Drug Administration as the source is appreciated but not required. | |
試験管内研究製品の免責事項と情報
BenchChemで提示されるすべての記事および製品情報は、情報提供を目的としています。BenchChemで購入可能な製品は、生体外研究のために特別に設計されています。生体外研究は、ラテン語の "in glass" に由来し、生物体の外で行われる実験を指します。これらの製品は医薬品または薬として分類されておらず、FDAから任何の医療状態、病気、または疾患の予防、治療、または治癒のために承認されていません。これらの製品を人間または動物に体内に導入する形態は、法律により厳格に禁止されています。これらのガイドラインに従うことは、研究と実験において法的および倫理的な基準の遵守を確実にするために重要です。