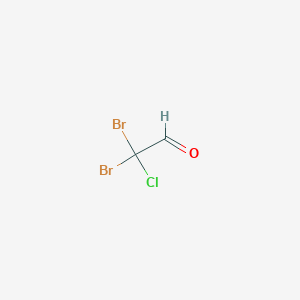
クロロジブロモアセトアルデヒド
説明
Chlorodibromoacetaldehyde, also known as Chlorodibromoacetaldehyde, is a useful research compound. Its molecular formula is C2HBr2ClO and its molecular weight is 236.29 g/mol. The purity is usually 95%.
BenchChem offers high-quality Chlorodibromoacetaldehyde suitable for many research applications. Different packaging options are available to accommodate customers' requirements. Please inquire for more information about Chlorodibromoacetaldehyde including the price, delivery time, and more detailed information at info@benchchem.com.
科学的研究の応用
. したがって、飲用水系におけるその安定性と挙動を理解することは重要です。
水と廃水処理における吸着剤
クロロジブロモアセトアルデヒドは、水と廃水処理において吸着剤として使用される可能性があります。 研究者らは、持続可能な用途のための低コストの吸着剤を探求しており、この化合物は水溶液から汚染物質を除去する際に役割を果たす可能性があります .
材料開発:セラミックスと航空宇宙
クロロジブロモアセトアルデヒドを含む臭素化化合物を含むセラミックスは、極端な温度と過酷な条件に耐える能力があるため、航空宇宙および防衛分野で用途が見られます。 これらの材料は、ヒートシールドや保護コーティングなどの部品に最適です .
アトロプ異性体合成
最近の研究では、官能化アトロプ異性体の前駆体である光学活性な2,2'-ジブロモ-6,6'-ジヨード-1,1'-ビフェニルの合成が強調されています。この化合物は、2 つの C—Br 結合と 2 つの C—I 結合を有しています。 これは、カルボキシル化、ボリル化、酸化、ホスフィニル化など、さまざまな官能基を形成するために選択的に進行させることができ、さまざまな官能化された軸不斉ビフェニルにつながります .
殺生物剤用途
広く研究されていませんが、クロロジブロモアセトアルデヒドの殺生物剤としての可能性は、調査する価値があります。 その迅速な化学的分解と抗菌活性により、トウモロコシからエタノールへの発酵などの工業プロセスにおける細菌制御など、さまざまな用途において興味深い候補となります .
作用機序
Target of Action
The primary targets of Chlorodibromoacetaldehyde are currently unknown. The compound’s interaction with its targets is a crucial aspect of its mechanism of action . The identification of these targets would provide valuable insights into the compound’s therapeutic potential .
Mode of Action
Understanding the compound’s interaction with its targets and the resulting changes is essential for elucidating its mechanism of action . This involves studying how the compound binds to its targets and the subsequent effects of this binding .
Biochemical Pathways
The compound likely interacts with various biochemical pathways, leading to downstream effects . These effects could include alterations in metabolic processes, changes in cell signaling, or modifications to gene expression .
Pharmacokinetics
These properties significantly impact the compound’s bioavailability . Understanding the pharmacokinetics of Chlorodibromoacetaldehyde would provide insights into how the compound is processed in the body and how these processes affect its therapeutic efficacy .
Result of Action
The molecular and cellular effects of Chlorodibromoacetaldehyde’s action are currently unknown. These effects could include changes in cellular function, alterations in molecular pathways, or modifications to gene expression . Understanding these effects is crucial for elucidating the compound’s mechanism of action and potential therapeutic applications .
Action Environment
The action of Chlorodibromoacetaldehyde is likely influenced by various environmental factors. These factors could include pH, temperature, and the presence of other molecules . For instance, the compound’s stability and efficacy could be affected by changes in pH or temperature . Understanding how these environmental factors influence the action of Chlorodibromoacetaldehyde could provide valuable insights into optimizing its use and improving its therapeutic efficacy.
特性
IUPAC Name |
2,2-dibromo-2-chloroacetaldehyde | |
---|---|---|
Source | PubChem | |
URL | https://pubchem.ncbi.nlm.nih.gov | |
Description | Data deposited in or computed by PubChem | |
InChI |
InChI=1S/C2HBr2ClO/c3-2(4,5)1-6/h1H | |
Source | PubChem | |
URL | https://pubchem.ncbi.nlm.nih.gov | |
Description | Data deposited in or computed by PubChem | |
InChI Key |
SFWAQPWRFZOPKA-UHFFFAOYSA-N | |
Source | PubChem | |
URL | https://pubchem.ncbi.nlm.nih.gov | |
Description | Data deposited in or computed by PubChem | |
Canonical SMILES |
C(=O)C(Cl)(Br)Br | |
Source | PubChem | |
URL | https://pubchem.ncbi.nlm.nih.gov | |
Description | Data deposited in or computed by PubChem | |
Molecular Formula |
C2HBr2ClO | |
Source | PubChem | |
URL | https://pubchem.ncbi.nlm.nih.gov | |
Description | Data deposited in or computed by PubChem | |
DSSTOX Substance ID |
DTXSID6021531 | |
Record name | Chlorodibromoacetaldehyde | |
Source | EPA DSSTox | |
URL | https://comptox.epa.gov/dashboard/DTXSID6021531 | |
Description | DSSTox provides a high quality public chemistry resource for supporting improved predictive toxicology. | |
Molecular Weight |
236.29 g/mol | |
Source | PubChem | |
URL | https://pubchem.ncbi.nlm.nih.gov | |
Description | Data deposited in or computed by PubChem | |
CAS No. |
64316-11-6 | |
Record name | Acetaldehyde, 2,2-dibromo-2-chloro- | |
Source | ChemIDplus | |
URL | https://pubchem.ncbi.nlm.nih.gov/substance/?source=chemidplus&sourceid=0064316116 | |
Description | ChemIDplus is a free, web search system that provides access to the structure and nomenclature authority files used for the identification of chemical substances cited in National Library of Medicine (NLM) databases, including the TOXNET system. | |
Retrosynthesis Analysis
AI-Powered Synthesis Planning: Our tool employs the Template_relevance Pistachio, Template_relevance Bkms_metabolic, Template_relevance Pistachio_ringbreaker, Template_relevance Reaxys, Template_relevance Reaxys_biocatalysis model, leveraging a vast database of chemical reactions to predict feasible synthetic routes.
One-Step Synthesis Focus: Specifically designed for one-step synthesis, it provides concise and direct routes for your target compounds, streamlining the synthesis process.
Accurate Predictions: Utilizing the extensive PISTACHIO, BKMS_METABOLIC, PISTACHIO_RINGBREAKER, REAXYS, REAXYS_BIOCATALYSIS database, our tool offers high-accuracy predictions, reflecting the latest in chemical research and data.
Strategy Settings
Precursor scoring | Relevance Heuristic |
---|---|
Min. plausibility | 0.01 |
Model | Template_relevance |
Template Set | Pistachio/Bkms_metabolic/Pistachio_ringbreaker/Reaxys/Reaxys_biocatalysis |
Top-N result to add to graph | 6 |
Feasible Synthetic Routes
Q1: What is notable about the polymerization of chlorodibromoacetaldehyde according to the research?
A1: The research paper states that chlorodibromoacetaldehyde, when polymerized, yields polymers that are completely insoluble. [] Furthermore, the resulting polymers are seemingly isotactic in nature. [] This suggests a high degree of structural regularity within the polymer chains formed.
Q2: The tribromomethyl group of bromal is mentioned as being significant. How does the side group of chlorodibromoacetaldehyde compare?
A2: The research highlights that bromal's tribromomethyl group is the largest successfully polymerized to date. [] While not explicitly stated, the successful polymerization of chlorodibromoacetaldehyde implies its dichlorobromomethyl side group, though smaller, still presents a significant steric challenge in polymerization. This characteristic likely contributes to the observed insolubility of the resulting polymer.
試験管内研究製品の免責事項と情報
BenchChemで提示されるすべての記事および製品情報は、情報提供を目的としています。BenchChemで購入可能な製品は、生体外研究のために特別に設計されています。生体外研究は、ラテン語の "in glass" に由来し、生物体の外で行われる実験を指します。これらの製品は医薬品または薬として分類されておらず、FDAから任何の医療状態、病気、または疾患の予防、治療、または治癒のために承認されていません。これらの製品を人間または動物に体内に導入する形態は、法律により厳格に禁止されています。これらのガイドラインに従うことは、研究と実験において法的および倫理的な基準の遵守を確実にするために重要です。