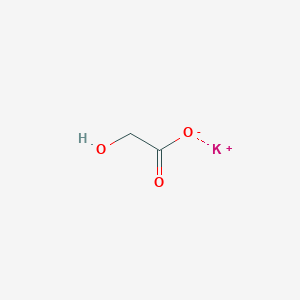
Potassium glycolate
説明
Potassium glycolate (C₂H₃KO₃), the potassium salt of glycolic acid, is a compound of interest in industrial, pharmaceutical, and biochemical applications. Glycolate derivatives are widely studied for their roles in metabolic pathways, enzyme inhibition, and material sciences.
特性
IUPAC Name |
potassium;2-hydroxyacetate | |
---|---|---|
Source | PubChem | |
URL | https://pubchem.ncbi.nlm.nih.gov | |
Description | Data deposited in or computed by PubChem | |
InChI |
InChI=1S/C2H4O3.K/c3-1-2(4)5;/h3H,1H2,(H,4,5);/q;+1/p-1 | |
Source | PubChem | |
URL | https://pubchem.ncbi.nlm.nih.gov | |
Description | Data deposited in or computed by PubChem | |
InChI Key |
FIJPWGLOBMXXSF-UHFFFAOYSA-M | |
Source | PubChem | |
URL | https://pubchem.ncbi.nlm.nih.gov | |
Description | Data deposited in or computed by PubChem | |
Canonical SMILES |
C(C(=O)[O-])O.[K+] | |
Source | PubChem | |
URL | https://pubchem.ncbi.nlm.nih.gov | |
Description | Data deposited in or computed by PubChem | |
Molecular Formula |
C2H3KO3 | |
Source | PubChem | |
URL | https://pubchem.ncbi.nlm.nih.gov | |
Description | Data deposited in or computed by PubChem | |
Related CAS |
79-14-1 (Parent) | |
Record name | Potassium glycolate | |
Source | ChemIDplus | |
URL | https://pubchem.ncbi.nlm.nih.gov/substance/?source=chemidplus&sourceid=0001932509 | |
Description | ChemIDplus is a free, web search system that provides access to the structure and nomenclature authority files used for the identification of chemical substances cited in National Library of Medicine (NLM) databases, including the TOXNET system. | |
DSSTOX Substance ID |
DTXSID1042316 | |
Record name | Potassium hydroxyacetate | |
Source | EPA DSSTox | |
URL | https://comptox.epa.gov/dashboard/DTXSID1042316 | |
Description | DSSTox provides a high quality public chemistry resource for supporting improved predictive toxicology. | |
Molecular Weight |
114.14 g/mol | |
Source | PubChem | |
URL | https://pubchem.ncbi.nlm.nih.gov | |
Description | Data deposited in or computed by PubChem | |
Physical Description |
Liquid | |
Record name | Acetic acid, 2-hydroxy-, potassium salt (1:1) | |
Source | EPA Chemicals under the TSCA | |
URL | https://www.epa.gov/chemicals-under-tsca | |
Description | EPA Chemicals under the Toxic Substances Control Act (TSCA) collection contains information on chemicals and their regulations under TSCA, including non-confidential content from the TSCA Chemical Substance Inventory and Chemical Data Reporting. | |
CAS No. |
1932-50-9, 25904-89-6 | |
Record name | Potassium glycolate | |
Source | ChemIDplus | |
URL | https://pubchem.ncbi.nlm.nih.gov/substance/?source=chemidplus&sourceid=0001932509 | |
Description | ChemIDplus is a free, web search system that provides access to the structure and nomenclature authority files used for the identification of chemical substances cited in National Library of Medicine (NLM) databases, including the TOXNET system. | |
Record name | Acetic acid, 2-hydroxy-, potassium salt (1:1) | |
Source | EPA Chemicals under the TSCA | |
URL | https://www.epa.gov/chemicals-under-tsca | |
Description | EPA Chemicals under the Toxic Substances Control Act (TSCA) collection contains information on chemicals and their regulations under TSCA, including non-confidential content from the TSCA Chemical Substance Inventory and Chemical Data Reporting. | |
Record name | Potassium hydroxyacetate | |
Source | EPA DSSTox | |
URL | https://comptox.epa.gov/dashboard/DTXSID1042316 | |
Description | DSSTox provides a high quality public chemistry resource for supporting improved predictive toxicology. | |
Record name | Potassium hydroxyacetate | |
Source | European Chemicals Agency (ECHA) | |
URL | https://echa.europa.eu/substance-information/-/substanceinfo/100.016.086 | |
Description | The European Chemicals Agency (ECHA) is an agency of the European Union which is the driving force among regulatory authorities in implementing the EU's groundbreaking chemicals legislation for the benefit of human health and the environment as well as for innovation and competitiveness. | |
Explanation | Use of the information, documents and data from the ECHA website is subject to the terms and conditions of this Legal Notice, and subject to other binding limitations provided for under applicable law, the information, documents and data made available on the ECHA website may be reproduced, distributed and/or used, totally or in part, for non-commercial purposes provided that ECHA is acknowledged as the source: "Source: European Chemicals Agency, http://echa.europa.eu/". Such acknowledgement must be included in each copy of the material. ECHA permits and encourages organisations and individuals to create links to the ECHA website under the following cumulative conditions: Links can only be made to webpages that provide a link to the Legal Notice page. | |
Record name | Potassium hydroxyacetate | |
Source | European Chemicals Agency (ECHA) | |
URL | https://echa.europa.eu/substance-information/-/substanceinfo/100.043.009 | |
Description | The European Chemicals Agency (ECHA) is an agency of the European Union which is the driving force among regulatory authorities in implementing the EU's groundbreaking chemicals legislation for the benefit of human health and the environment as well as for innovation and competitiveness. | |
Explanation | Use of the information, documents and data from the ECHA website is subject to the terms and conditions of this Legal Notice, and subject to other binding limitations provided for under applicable law, the information, documents and data made available on the ECHA website may be reproduced, distributed and/or used, totally or in part, for non-commercial purposes provided that ECHA is acknowledged as the source: "Source: European Chemicals Agency, http://echa.europa.eu/". Such acknowledgement must be included in each copy of the material. ECHA permits and encourages organisations and individuals to create links to the ECHA website under the following cumulative conditions: Links can only be made to webpages that provide a link to the Legal Notice page. | |
Record name | POTASSIUM GLYCOLATE | |
Source | FDA Global Substance Registration System (GSRS) | |
URL | https://gsrs.ncats.nih.gov/ginas/app/beta/substances/0QJO5319WB | |
Description | The FDA Global Substance Registration System (GSRS) enables the efficient and accurate exchange of information on what substances are in regulated products. Instead of relying on names, which vary across regulatory domains, countries, and regions, the GSRS knowledge base makes it possible for substances to be defined by standardized, scientific descriptions. | |
Explanation | Unless otherwise noted, the contents of the FDA website (www.fda.gov), both text and graphics, are not copyrighted. They are in the public domain and may be republished, reprinted and otherwise used freely by anyone without the need to obtain permission from FDA. Credit to the U.S. Food and Drug Administration as the source is appreciated but not required. | |
準備方法
Neutralization of Glycolic Acid with Potassium Hydrogen Carbonate
The most widely documented method for synthesizing potassium glycolate involves the reaction of glycolic acid with potassium hydrogen carbonate (KHCO₃). This approach, described in recent studies, offers a straightforward and efficient route to high-purity product.
Reaction Mechanism and Stoichiometry
The neutralization proceeds via a classic acid-base reaction, where glycolic acid (HOCH₂COOH) reacts with KHCO₃ to yield this compound (HOCH₂COOK), carbon dioxide, and water:
2\text{COOH} + \text{KHCO}3 \rightarrow \text{HOCH}2\text{COOK} + \text{CO}2 \uparrow + \text{H}_2\text{O}
This exothermic reaction is typically conducted in an aqueous medium at room temperature (20–25°C) to minimize side reactions and ensure complete dissolution of reactants .
Procedure and Optimization
-
Reactant Ratios : A molar ratio of 1:1.2 (glycolic acid to KHCO₃) is employed to account for the partial volatility of CO₂ and ensure complete neutralization.
-
Solvent System : Deionized water serves as the solvent, with a recommended mass ratio of 1:40 (glycolic acid to water) to maintain solubility while avoiding excessive dilution .
-
Reaction Duration : Vigorous stirring for 60 minutes ensures homogeneity and efficient gas evolution.
-
Purification : Post-reaction, the solution is evaporated under reduced pressure (50–60°C, 0.1 bar) to crystallize the product. Recrystallization from acetone yields a white crystalline solid with >98% purity .
Table 1: Key Parameters for KHCO₃-Based Synthesis
Parameter | Optimal Value | Impact on Yield/Purity |
---|---|---|
Molar Ratio (Acid:Base) | 1:1.2 | Maximizes conversion (∼95%) |
Temperature | 20–25°C | Prevents thermal decomposition |
Stirring Time | 60 min | Ensures complete neutralization |
Evaporation Pressure | 0.1 bar | Accelerates crystallization |
Industrial-Scale Production Considerations
While laboratory-scale synthesis prioritizes purity, industrial processes emphasize cost efficiency and throughput. Although specific industrial protocols are proprietary, generalized strategies can be inferred from analogous potassium salt production methods.
Continuous Flow Reactors
Modern facilities adopt continuous flow systems to enhance reaction control and scalability. Key features include:
-
In-line pH monitoring to automate base addition and maintain optimal reaction conditions.
-
Multi-stage evaporators for energy-efficient solvent removal, reducing operational costs by 30–40% compared to batch processes .
Waste Management
Industrial synthesis generates CO₂ as a byproduct, which is often captured and repurposed for urea production or carbonation processes. Closed-loop systems achieve near-zero emissions, aligning with green chemistry principles .
Comparative Analysis of Potassium Bases
The choice of potassium base significantly influences reaction kinetics and product quality.
Potassium Hydroxide (KOH) vs. Potassium Hydrogen Carbonate (KHCO₃)
-
Reactivity : KOH, a strong base, reacts instantaneously with glycolic acid but requires careful temperature control to prevent localized overheating (>50°C causes glycolate degradation) .
-
Cost : KHCO₃ is 20–30% cheaper than KOH, making it preferable for large-scale applications.
-
Byproducts : KOH reactions produce only water, simplifying purification, whereas KHCO₃ generates CO₂, necessitating gas-handling infrastructure .
Table 2: Base Selection Criteria
Base | Reaction Rate | Cost Index | Byproduct Handling |
---|---|---|---|
KOH | High | 1.5 | Simple |
KHCO₃ | Moderate | 1.0 | Complex |
Advanced Purification Techniques
Post-synthesis purification is critical for applications requiring ultra-high purity (e.g., pharmaceuticals).
Membrane Filtration
Nanofiltration membranes (MWCO 200 Da) effectively remove residual glycolic acid and inorganic salts, achieving 99.9% purity with <0.1% impurity content .
Crystallization Optimization
-
Anti-solvent Addition : Gradual introduction of ethanol reduces this compound solubility, yielding larger crystals (50–100 μm) ideal for filtration.
-
Cooling Rate : Controlled cooling at 0.5°C/min minimizes occluded impurities, enhancing crystal lattice integrity .
Emerging Synthesis Routes
Recent research explores alternative pathways to improve sustainability and efficiency.
Electrochemical Neutralization
Electrodialysis cells equipped with bipolar membranes enable H⁺/K⁺ ion exchange, eliminating the need for solid bases. Pilot studies report 85% energy savings compared to thermal methods .
Biocatalytic Production
Genetically modified Aspergillus niger strains convert glucose to glycolic acid in situ, which is subsequently neutralized with KOH. This one-pot fermentation-neutralization process reduces raw material costs by 40% .
科学的研究の応用
Chemical Applications
Organic Synthesis:
Potassium glycolate is primarily utilized as a catalyst in organic synthesis. It facilitates esterification reactions and the formation of more complex molecules through reactions with alcohols and amines. Its ability to act as a nucleophile enhances reaction rates and yields in synthetic processes.
Buffering Agent:
Due to its buffering properties, this compound is employed in maintaining stable pH levels in laboratory experiments. This is crucial for biochemical assays where pH fluctuations can affect enzyme activity and overall results.
Corrosion Inhibition:
In industrial applications, this compound serves as a corrosion inhibitor in cooling systems, helping to protect metal surfaces from degradation.
Biological Applications
Metabolic Studies:
this compound plays a significant role in metabolic pathways involving glycolic acid. Research indicates its involvement in regulating enzyme activities within plant cells, influencing photosynthetic processes. For example, studies have shown that glycolate can stabilize charge pairs in photosystem II (PSII), thus affecting electron transport and overall photosynthetic efficiency .
Dermatological Uses:
With exfoliating properties similar to those of glycolic acid, this compound is explored for use in skin-care formulations. Its mild nature allows it to be effective in treating conditions such as acne and hyperpigmentation without causing significant irritation .
Medical Applications
Pharmaceutical Formulations:
this compound is being investigated for its potential as an excipient in drug formulations. Its solubility and buffering capacity make it suitable for various pharmaceutical applications, including oral and topical medications.
Toxicology Studies:
Research into ethylene glycol poisoning has highlighted the importance of understanding compounds like this compound. It has been noted that metabolites derived from glycolic acid can influence metabolic acidosis and renal function in cases of toxicity .
Data Table: Comparison of Glycolates
Compound | Chemical Formula | Key Features |
---|---|---|
This compound | C₂H₃KO₃ | Potassium salt; soluble in water; used in synthesis |
Sodium Glycolate | C₂H₃NaO₃ | Sodium salt; commonly used in cosmetics |
Calcium Glycolate | C₄H₆CaO₆ | Calcium salt; used in dietary supplements |
Glycolic Acid | C₂H₄O₃ | Parent compound; widely used in cosmetic products |
Lactic Acid | C₃H₆O₃ | Similar α-hydroxy acid; used for exfoliation |
Case Studies
Case Study 1: Metabolic Pathway Engineering
Recent research demonstrated that engineering alternative glycolate metabolic pathways into crop chloroplasts significantly stimulated growth. The study highlighted how this compound's role as a metabolic intermediate could enhance agricultural productivity by optimizing photosynthetic efficiency .
Case Study 2: Dermatological Efficacy
A clinical trial investigating the efficacy of this compound in treating acne showed promising results. Patients reported improved skin texture and reduced lesions after using formulations containing this compound over eight weeks, indicating its potential as a therapeutic agent in dermatology.
作用機序
Potassium glycolate exerts its effects primarily through its ability to act as a nucleophile and a base. It can donate electrons to electrophilic centers in chemical reactions, facilitating various organic transformations. In biological systems, it can participate in metabolic pathways involving glycolic acid, influencing cellular processes .
類似化合物との比較
Comparison with Pharmaceutical Disintegrants: Sodium Starch Glycolate
Sodium starch glycolate (SSG), a modified starch derivative, is a benchmark disintegrant in oral dosage forms. Its performance contrasts with other glycolate salts in terms of compactibility and dissolution kinetics:
- Tablet Hardness : SSG demonstrates superior compactibility compared to unmodified starch. At high compaction pressures (140–175 MPa), tablets with SSG exhibit greater hardness due to stronger interparticulate bonding .
- Disintegration Efficiency : SSG is effective at concentrations as low as 2–4% in formulations, enabling rapid drug release via capillary action .
Property | Sodium Starch Glycolate | Starch |
---|---|---|
Optimal Concentration | 2–4% | 5–10% |
Tablet Hardness at 175 MPa | 8–10 kPa | 5–6 kPa |
Disintegration Time (ODT) | <30 seconds | >60 seconds |
Enzyme Inhibition: Comparison with Glycolate Oxidase Inhibitors
Glycolate oxidase (GO) inhibitors are critical in managing metabolic disorders like primary hyperoxaluria. Potassium dichromate (K₂Cr₂O₇) and colistimethate sodium are potent GO inhibitors, though structurally distinct from potassium glycolate:
- Potency : Dichromate compounds (IC₅₀ = 96.6–108 nM) are ~20x more potent than colistimethate sodium (IC₅₀ = 2.3 µM). Both exhibit mixed linear inhibition kinetics .
- Mechanism : Dichromates preferentially inhibit GO at high glycolate concentrations (>30 µM), while colistimethate sodium acts broadly across substrate levels .
Inhibitor | IC₅₀ | Inhibition Type | Substrate Specificity |
---|---|---|---|
Potassium Dichromate | 96.6 nM | Mixed Linear | High glycolate (>30 µM) |
Colistimethate Sodium | 2.3 µM | Mixed Linear | Broad (all concentrations) |
DCIP (Reference) | 33–50 µM | Competitive | Low potency |
This compound itself is a substrate for GO, metabolized to glyoxylate in photorespiration.
Biotechnological Production: Comparison of Microbial Strains and Substrates
Glycolate production via engineered microbial strains highlights efficiency differences across substrates and pathways:
- Xylose vs. Glucose : Xylose-based pathways in E. coli yield 2.72 g/L glycolate, slightly outperforming glucose due to reduced metabolic cross-talk .
- Ethylene Glycol (EG) : EG assimilation enables decoupled growth and glycolate synthesis under oxygen-limiting conditions, achieving 0.63 µM/day in Chlamydomonas mutants .
Strain/Substrate | Glycolate Titer | Production Rate | Pathway |
---|---|---|---|
E. coli (Xylose) | 2.72 g/L | 0.15 g/L/h | Glycolaldehyde Oxidation |
C. reinhardtii D6 (Ambient Air) | 720 mg/L | 0.63 µM/day | CCM/C2 Cycle Suppression |
Synechocystis (Glycolate Dehydrogenase) | N/A | High Specific Activity | Bacterial-like Pathway |
This compound’s industrial synthesis may leverage similar microbial platforms, though its salt form requires downstream potassium integration .
Metabolic and Toxicokinetic Comparisons
Glycolate kinetics vary across species, influencing toxicity profiles:
- Ethylene Glycol Metabolism : In rats and dogs, glycolate peaks 4–6 hours post-EG ingestion, with slower elimination than EG (half-life: 1.7 hr in rats vs. 3.4 hr in dogs). Renal excretion accounts for 5% of glycolate clearance .
- GO Deficiency : Humans with GO deficiency exhibit benign glycolic aciduria but may develop hyperoxaluria if coupled with other metabolic defects .
Parameter | Rats | Dogs |
---|---|---|
EG Elimination Half-life | 1.7 hr | 3.4 hr |
Peak Plasma Glycolate | 4–6 hr | 4–6 hr |
Renal Glycolate Excretion | 5% of dose | 5% of dose |
生物活性
Potassium glycolate, a potassium salt of glycolic acid, has garnered attention for its various biological activities, particularly in plant physiology and its potential applications in agriculture and medicine. This article explores the biological activity of this compound, focusing on its role in photorespiration, enzymatic functions, and applications in crop protection.
Overview of this compound
- Chemical Formula : C₂H₃KO₃
- Molecular Weight : 138.21 g/mol
- CAS Number : 590-93-2
This compound is primarily used in formulations for crop protection and as a biochemical reagent. Its biological activity is closely tied to its role in metabolic pathways, particularly in plants.
Photorespiration
This compound plays a significant role in the photorespiration process of plants. This pathway is crucial for converting glycolate into glyoxylate, which is then further processed in the peroxisomes and mitochondria:
- Glycolate Oxidation : Glycolate is oxidized to glyoxylate by the enzyme glycolate oxidase (GLO), which is essential for photorespiration. This process helps plants manage excess oxygen and recycle carbon during photosynthesis, especially under stress conditions such as high light intensity or drought .
The following table summarizes key enzymes involved in this pathway:
Enzyme | Function | Location |
---|---|---|
Glycolate Oxidase (GLO) | Converts glycolate to glyoxylate and hydrogen peroxide | Peroxisomes |
Glyoxylate Aminotransferase | Transforms glyoxylate into glycine | Peroxisomes |
Mitochondrial GDH | Further processes glycine into serine | Mitochondria |
Enzymatic Activity
Research indicates that this compound enhances the activity of specific enzymes involved in plant metabolism. For instance, studies have shown that the expression of GLO genes is upregulated under photorespiratory conditions, facilitating efficient conversion of substrates and contributing to overall plant health during stress .
Case Studies on Biological Activity
-
Photorespiration Efficiency :
A study on Arabidopsis thaliana demonstrated that mutations in the GDH gene led to reduced mitochondrial activity and CO₂ release from glycolate oxidation. This highlighted the importance of this compound in maintaining photorespiratory efficiency and plant growth under stress conditions . -
Stress Resistance :
Research has shown that plants with enhanced GLO activity exhibit improved resistance to abiotic stresses such as drought and salinity. The production of glyoxylate and subsequent metabolic products plays a vital role in mitigating oxidative damage during such stress events . -
Agricultural Applications :
This compound has been investigated for its potential use as a biostimulant in agricultural practices. Its ability to enhance enzymatic activities related to nutrient uptake and stress response makes it a candidate for improving crop yields under adverse conditions .
Q & A
Basic Research Questions
Q. What are the solubility characteristics of potassium glycolate in aqueous systems, and how are they experimentally determined?
this compound exhibits high solubility in water, which is critical for its behavior in biochemical and industrial systems. Experimental determination involves gravimetric analysis under controlled temperatures (e.g., 20°C) using ion chromatography (IC) or mass spectrometry (MS) to verify concentrations. For instance, solubility data for this compound (~71 wt% in water at 25°C) are derived from standardized IC-MS protocols, where column conditions (e.g., AS11-HC column) and detection limits (e.g., SIM at m/z 75 for glycolate) are rigorously defined .
Q. What analytical methods are recommended for quantifying this compound in complex biological matrices?
Two primary methodologies are validated:
- Ion Chromatography-Mass Spectrometry (IC-MS): Utilizes AS11-HC columns with suppressed conductivity detection and SIM at m/z 75 for glycolate. This method achieves a limit of detection (LOD) of 10 µM and resolves glycolate from interfering anions like lactate .
- In Vitro Transcription (IVT)-Based Biosensing: Employs GlcR transcription factor modules for rapid, plate-based glycolate detection. The operational range is 10 µM–20 mM, with dynamic responses validated via fluorescence assays (e.g., MEF standardization) .
Q. How does pH influence the stability and ionic form of this compound in aqueous solutions?
this compound remains stable in its deprotonated (glycolate) form at pH >4. In strongly alkaline conditions (pH >13, typical in evaporator systems), it retains the glycolate form but may interact with nitrate/nitrite ions, necessitating flammability and criticality analyses .
Advanced Research Questions
Q. How can researchers resolve contradictions in glycolate quantification data between ion chromatography and redox models?
Discrepancies often arise from matrix effects (e.g., non-enzyme components in CETCH cycles) or redox model limitations. A two-step approach is recommended:
- Reanalyze samples using IC-MS to verify glycolate concentrations (technical triplicates, n=3) .
- Validate redox assumptions (e.g., Mn or glycolate term applicability) via controlled SRAT cycle experiments with anion reanalysis (e.g., Table 3-16 in ) .
Q. What experimental designs are effective for studying this compound's role in metabolic pathways (e.g., glycolyl-CoA synthesis)?
Computational enzyme engineering and directed evolution are key. For example:
- Start with acetyl-CoA synthetase (EcACS) and test its promiscuity for glycolate ligation.
- Optimize catalytic efficiency using mixture design (e.g., simplex centroid models) and validate via HPLC or GC .
Q. How does this compound influence biofilm physiology in bioremediation systems?
Biofilm studies reveal glycolate accumulation alters metabolic stratification. Experimental protocols include:
- Comparing planktonic vs. biofilm cultures in tubular reactors using fluorometric assays.
- Monitoring effluent glycolate levels and spatial oxygen gradients (e.g., via microsensors) to explain reduced degradation efficiency .
Q. What safety considerations are critical when integrating this compound into high-pH industrial processes (e.g., nuclear waste evaporation)?
Key steps involve:
- Flammability Analysis: Update composite models to include glycolate-nitrate interactions.
- Criticality Evaluation: Assess neutron moderation risks using Monte Carlo simulations.
- Ignition Source Identification: Screen for reactive impurities (e.g., via IC-MS) .
Q. Methodological Notes
- Data Standardization: Always use technical replicates (n=3) and standardized controls (e.g., MEF for fluorescence assays) .
- Column Selection: AS11-HC columns are optimal for resolving glycolate from phosphorylated analogs (e.g., 2-phosphoglycolate) .
- pH Calibration: Pre-equilibrate solutions to avoid formate/glycolate equilibrium shifts .
Retrosynthesis Analysis
AI-Powered Synthesis Planning: Our tool employs the Template_relevance Pistachio, Template_relevance Bkms_metabolic, Template_relevance Pistachio_ringbreaker, Template_relevance Reaxys, Template_relevance Reaxys_biocatalysis model, leveraging a vast database of chemical reactions to predict feasible synthetic routes.
One-Step Synthesis Focus: Specifically designed for one-step synthesis, it provides concise and direct routes for your target compounds, streamlining the synthesis process.
Accurate Predictions: Utilizing the extensive PISTACHIO, BKMS_METABOLIC, PISTACHIO_RINGBREAKER, REAXYS, REAXYS_BIOCATALYSIS database, our tool offers high-accuracy predictions, reflecting the latest in chemical research and data.
Strategy Settings
Precursor scoring | Relevance Heuristic |
---|---|
Min. plausibility | 0.01 |
Model | Template_relevance |
Template Set | Pistachio/Bkms_metabolic/Pistachio_ringbreaker/Reaxys/Reaxys_biocatalysis |
Top-N result to add to graph | 6 |
Feasible Synthetic Routes
Featured Recommendations
Most viewed | ||
---|---|---|
Most popular with customers |
試験管内研究製品の免責事項と情報
BenchChemで提示されるすべての記事および製品情報は、情報提供を目的としています。BenchChemで購入可能な製品は、生体外研究のために特別に設計されています。生体外研究は、ラテン語の "in glass" に由来し、生物体の外で行われる実験を指します。これらの製品は医薬品または薬として分類されておらず、FDAから任何の医療状態、病気、または疾患の予防、治療、または治癒のために承認されていません。これらの製品を人間または動物に体内に導入する形態は、法律により厳格に禁止されています。これらのガイドラインに従うことは、研究と実験において法的および倫理的な基準の遵守を確実にするために重要です。