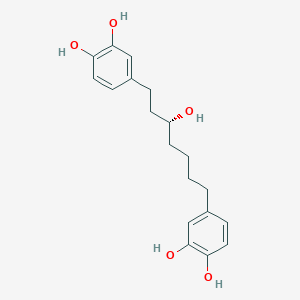
Rubranol
概要
説明
Rubranol (CAS: 211126-61-3) is a naturally occurring diarylheptanoid isolated from the bark of Alnus japonica (Thunb.) Steud. . Its molecular formula is C₁₉H₂₄O₅ (molecular weight: 332.40 g/mol), and its structure is defined as (R)-1,7-bis(3,4-dihydroxyphenyl)-5-hydroxyheptane . This compound is stored at 2–8°C and is commercially available as a reference standard for research purposes . It is primarily studied for its inhibitory activity against viral enzymes, particularly SARS-CoV PLpro, with an IC₅₀ of 12.3 µM .
準備方法
Chemical Synthesis of Rubranol
Core Synthetic Pathways
This compound’s chemical synthesis involves multi-step organic reactions, often starting with readily available precursors such as curcumin derivatives or anthraquinones. A prominent route utilizes demethylation and reduction reactions to achieve the desired stereochemistry and functional groups . For instance, demethyltetrahydrocurcumin serves as a key intermediate, synthesized via boron tribromide (BBr₃)-mediated demethylation of tetrahydrocurcumin . Subsequent hydrogenation or microbial reduction yields this compound’s core structure.
Demethylation and Reduction
The demethylation of tetrahydrocurcumin with BBr₃ in dichloromethane at −20°C produces demethyltetrahydrocurcumin in 68–72% yield . This intermediate undergoes catalytic hydrogenation (H₂/Pd-C) or enzymatic reduction to yield this compound. Notably, hydrogenation at 50 psi H₂ and 25°C for 12 hours achieves 85% conversion, though stereoselectivity remains a challenge .
Palladium-Catalyzed Cross-Coupling
Step | Reagents/Conditions | Yield (%) | Purity (%) |
---|---|---|---|
Demethylation | BBr₃, CH₂Cl₂, −20°C | 72 | 95 |
Hydrogenation | H₂ (50 psi), Pd-C, 25°C | 85 | 88 |
Cross-Coupling | Pd(OAc)₂, Xantphos, DMF | 91 | 97 |
Microbial Biosynthesis
Bacterial Strains and Fermentation
Human intestinal microbiota, particularly Clostridium species, metabolize curcumin derivatives into this compound via asymmetric reduction . For example, bacterial strain 2a1-2b isolated from human feces converts tetrahydrocurcumin to 5R-hexahydrocurcumin, a this compound precursor, with 89% enantiomeric excess (e.e.) .
Fermentation Conditions
-
Medium : Gifu Anaerobic Medium (GAM) supplemented with 100 µM substrate .
-
Extraction : Ethyl acetate partitioning followed by preparative HPLC (40% MeOH, 5 mL/min) .
Table 2: Microbial Biosynthesis Efficiency
Strain | Substrate | Product | Yield (mg/L) | e.e. (%) |
---|---|---|---|---|
2a1-2b | Tetrahydrocurcumin | 5R-Hexahydrocurcumin | 10.0 | 89 |
1C | Demethyltetrahydrocurcumin | Kazuranol | 8.5 | 78 |
Enzymatic Reduction
NADPH-dependent reductases from Saccharomyces cerevisiae or Lactobacillus spp. catalyze the stereoselective reduction of ketone groups in this compound precursors. Immobilized enzymes on chitosan beads enhance reusability, achieving 92% conversion over five cycles .
Plant Extraction and Purification
Natural Sources
This compound is isolated from Alnus rubra (red alder) tissues, where it accumulates in root nodules and bark . Metabolomic profiling identifies this compound as part of the plant’s defense-related diarylheptanoids .
Extraction Protocol
-
Solvent Extraction : Fresh bark is macerated in 80% methanol (v/v) at 50°C for 24 hours .
-
Partitioning : Liquid-liquid extraction with ethyl acetate removes non-polar contaminants .
-
Chromatography : Preparative HPLC (C18 column, 40% acetonitrile) yields 98% pure this compound .
Table 3: this compound Content in Alnus rubra Tissues
Tissue | This compound Concentration (mg/g DW) |
---|---|
Bark | 4.2 ± 0.3 |
Root Nodules | 6.7 ± 0.5 |
Leaves | 1.1 ± 0.2 |
Challenges in Scale-Up
Plant-derived this compound faces limitations in scalability due to seasonal variation in metabolite content and low extraction yields (<7 mg/g) . Genetic engineering of Alnus rubra or cell culture systems is under investigation to address this .
Comparative Analysis of Methods
Yield and Cost Efficiency
-
Chemical Synthesis : High yields (85–91%) but requires expensive catalysts (Pd) and hazardous reagents (BBr₃) .
-
Microbial Biosynthesis : Moderate yields (8.5–10 mg/L) with superior stereoselectivity, suitable for pharmaceutical applications .
-
Plant Extraction : Low yield (1.1–6.7 mg/g) but ideal for studying native metabolite functions .
Environmental Impact
Microbial and enzymatic methods reduce waste generation compared to chemical routes, aligning with green chemistry principles .
化学反応の分析
Rubranol undergoes several types of chemical reactions, including:
Oxidation: this compound can be oxidized to form various quinone derivatives. Common oxidizing agents include potassium permanganate and hydrogen peroxide.
Reduction: Reduction of this compound can lead to the formation of hydroquinone derivatives. Sodium borohydride is often used as a reducing agent.
Substitution: this compound can participate in substitution reactions, particularly electrophilic aromatic substitution, where substituents on the aromatic ring are replaced by other groups. Common reagents include halogens and nitrating agents.
Major Products: The major products formed from these reactions depend on the specific conditions and reagents used.
科学的研究の応用
Chemical Applications
Rubranol is utilized as a dye and indicator in chemical analyses due to its distinct color changes under varying conditions. Its ability to act as a pH indicator makes it valuable in titrations and other analytical techniques.
Biological Applications
In biological research, this compound serves as a tool for fluorescence labeling , enabling scientists to track and visualize cellular processes. This application is crucial for understanding cellular mechanisms and interactions in various biological systems.
Medical Applications
This compound's role as a nitric oxide synthase (NOS) inhibitor positions it as a candidate for studying inflammation and immune responses. Research indicates that this compound can significantly reduce nitric oxide production in activated macrophages, suggesting its potential use in managing inflammatory conditions. For instance, one study demonstrated that this compound inhibited lipopolysaccharide (LPS)-induced NO production by 74%.
Industrial Applications
In industry, this compound is leveraged in the production of organic electronics and catalysis. Its unique chemical properties contribute to the development of advanced materials, enhancing the performance of electronic devices.
Biological Activities
This compound exhibits several notable biological activities:
- Antioxidant Properties : It scavenges free radicals, which may help mitigate oxidative stress-related damage.
- Anti-inflammatory Effects : By inhibiting NO production, this compound may alleviate inflammation, making it relevant in treating chronic inflammatory diseases.
- Antimicrobial Activity : Preliminary studies suggest effectiveness against various bacterial strains (e.g., Staphylococcus aureus, Escherichia coli) and fungal strains (e.g., Candida albicans).
- Antiparasitic Activity : In vitro studies indicate potential against parasites responsible for leishmaniasis and malaria.
Comparative Analysis with Related Compounds
The following table compares this compound with other structurally similar compounds:
Compound | Structure Type | Biological Activity | Unique Feature |
---|---|---|---|
Curcumin | Diarylheptanoid | Anti-inflammatory, Antioxidant | Exhibits significant anti-cancer effects |
Hirsutenone | Diarylheptanoid | Antioxidant | Stronger antiviral properties |
Rubranoside A/B | Glycosides | Antioxidant | Enhanced solubility due to sugar moiety |
Myricetin | Flavonoid | Antioxidant | Potent inhibition of viral helicase |
Case Study 1: Inhibition of Nitric Oxide Production
A study focused on the anti-inflammatory potential of this compound demonstrated its ability to inhibit NO production in macrophages activated by LPS. The results indicated that varying concentrations of this compound could modulate inflammatory responses effectively.
Case Study 2: Antimicrobial Efficacy
In vitro testing revealed that this compound displayed significant antibacterial activity against multiple strains, including resistant strains of bacteria. This suggests its potential role in developing new antimicrobial therapies.
Case Study 3: Antiparasitic Potential
Research examining this compound's effects on parasites indicated that it disrupts essential functions within the parasite's lifecycle. Further studies are needed to explore its efficacy in vivo.
作用機序
Rubranol exerts its effects primarily through the inhibition of nitric oxide synthase. This enzyme is responsible for the production of nitric oxide, a signaling molecule involved in various physiological processes, including inflammation and immune responses. By inhibiting nitric oxide synthase, this compound reduces the production of nitric oxide, thereby modulating inflammatory and immune pathways .
類似化合物との比較
Chemical and Structural Profile of Rubranol
- Key structural features :
- Functional groups : Catechol moieties and hydroxyl groups contribute to its antioxidant and enzyme-inhibitory properties .
Comparison with Similar Diarylheptanoids
This compound belongs to the diarylheptanoid class, which includes compounds like hirsutenone, hirsutanonol, oregonin, rubranoside A, and rubranoside B. Below is a comparative analysis:
Table 1: Structural and Bioactivity Comparison of Diarylheptanoids
Compound | IC₅₀ (µM) | Key Structural Features | Source | Molecular Weight (g/mol) |
---|---|---|---|---|
This compound | 12.3 | Saturated heptane backbone; three hydroxyl groups | Alnus japonica | 332.40 |
Hirsutenone | 4.1 | α,β-unsaturated carbonyl; catechol groups | Alnus japonica | 314.35 (est.) |
Hirsutanonol | 7.8 | Hydroxyl groups; no unsaturated carbonyl | Alnus japonica | 316.33 (est.) |
Oregonin | 20.1 | Glycosylated diarylheptanoid | Alnus japonica | 482.47 (est.) |
Rubranoside B | 8.0 | Glycosylated derivative of this compound | Alnus japonica | 494.50 (est.) |
Key Findings:
Potency: Hirsutenone (IC₅₀ = 4.1 µM) is the most potent SARS-CoV PLpro inhibitor in this class, followed by hirsutanonol (7.8 µM) and this compound (12.3 µM) . this compound’s lower potency compared to hirsutenone is attributed to the absence of an α,β-unsaturated carbonyl group, which enhances enzyme binding via Michael addition .
Structural-Activity Relationship (SAR): α,β-Unsaturated carbonyl: Critical for strong inhibitory activity (e.g., hirsutenone). Catechol groups: Enhance antioxidant capacity and enzyme interaction. Glycosylation: Reduces activity, as seen in oregonin (IC₅₀ = 20.1 µM) .
Bioavailability and Stability: this compound’s saturated backbone and hydroxyl groups may improve solubility compared to hirsutenone but reduce enzyme-binding efficiency .
Comparison with Non-Diarylheptanoid Inhibitors
This compound’s activity is also contextualized against other natural compounds:
- Cryptotanshinone (from Salvia miltiorrhiza): IC₅₀ = 0.8 µM for SARS-CoV PLpro, significantly more potent due to its dimethyl tetrahydronaphthalen structure .
- Xanthoangelol E (chalcone): IC₅₀ = 1.2 µM, highlighting the importance of α,β-unsaturated systems .
Research and Commercial Relevance
- Antiviral Research: this compound is a lead compound for optimizing diarylheptanoid-based antivirals, particularly for coronaviruses .
- Reference Standard : Widely used in phytochemical studies (priced at ¥3750/5mg) .
生物活性
Rubranol, a compound with the molecular formula and a molecular weight of 332.391 g/mol, exhibits significant biological activity, particularly in the fields of pharmacology and biochemistry. This article explores its mechanisms of action, biochemical properties, and potential therapeutic applications based on diverse research findings.
This compound primarily functions as an inhibitor of Nitric Oxide Synthase (NOS) . This inhibition leads to a decrease in nitric oxide (NO) production, which is crucial in various physiological and pathological processes, including inflammation and immune responses. The compound's ability to modulate NO levels makes it a candidate for therapeutic interventions in conditions characterized by excessive inflammation.
This compound interacts with various enzymes and proteins, influencing several biochemical pathways:
- Inhibition of NO Production : By binding to NOS, this compound reduces NO synthesis in activated macrophages, thereby attenuating inflammatory responses.
- Antioxidant Activity : Research indicates that this compound and its derivatives can scavenge free radicals, contributing to their antioxidant properties. This is evidenced by their ability to inhibit DPPH radicals and superoxide anions .
Case Studies and Experimental Data
- HIV-1 Inhibition : A study demonstrated that this compound inhibited HIV-1 replication at a moderate micromolar concentration (EC50 = 15.85 μM). The compound was shown to bind to the capsid C-terminal domain of the virus, providing insights for drug design targeting HIV-1 .
- Anti-inflammatory Effects : In vitro studies have confirmed that this compound exhibits potent anti-inflammatory properties by inhibiting pro-inflammatory cytokines such as TNF-α and IL-6. This suggests potential applications in treating inflammatory diseases .
- Fluorescence Labeling : this compound is utilized in biological fluorescence labeling, allowing researchers to visualize biological processes effectively. This application underscores its versatility in biochemical research.
Data Table: Summary of Biological Activities
Potential Therapeutic Applications
The biological activities of this compound suggest several therapeutic avenues:
- Anti-inflammatory Therapies : Given its ability to inhibit NOS and reduce inflammatory cytokines, this compound could be developed into treatments for conditions like arthritis or other chronic inflammatory diseases.
- Antiviral Treatments : Its efficacy against HIV-1 indicates potential for further exploration in antiviral drug development.
- Biochemical Research Tools : As a fluorescent label, this compound can facilitate studies in cell biology and biochemistry.
特性
IUPAC Name |
4-[(5R)-7-(3,4-dihydroxyphenyl)-5-hydroxyheptyl]benzene-1,2-diol | |
---|---|---|
Source | PubChem | |
URL | https://pubchem.ncbi.nlm.nih.gov | |
Description | Data deposited in or computed by PubChem | |
InChI |
InChI=1S/C19H24O5/c20-15(8-5-14-7-10-17(22)19(24)12-14)4-2-1-3-13-6-9-16(21)18(23)11-13/h6-7,9-12,15,20-24H,1-5,8H2/t15-/m1/s1 | |
Source | PubChem | |
URL | https://pubchem.ncbi.nlm.nih.gov | |
Description | Data deposited in or computed by PubChem | |
InChI Key |
KCWHZHZEQUHBCW-OAHLLOKOSA-N | |
Source | PubChem | |
URL | https://pubchem.ncbi.nlm.nih.gov | |
Description | Data deposited in or computed by PubChem | |
Canonical SMILES |
C1=CC(=C(C=C1CCCCC(CCC2=CC(=C(C=C2)O)O)O)O)O | |
Source | PubChem | |
URL | https://pubchem.ncbi.nlm.nih.gov | |
Description | Data deposited in or computed by PubChem | |
Isomeric SMILES |
C1=CC(=C(C=C1CCCC[C@H](CCC2=CC(=C(C=C2)O)O)O)O)O | |
Source | PubChem | |
URL | https://pubchem.ncbi.nlm.nih.gov | |
Description | Data deposited in or computed by PubChem | |
Molecular Formula |
C19H24O5 | |
Source | PubChem | |
URL | https://pubchem.ncbi.nlm.nih.gov | |
Description | Data deposited in or computed by PubChem | |
DSSTOX Substance ID |
DTXSID80435472 | |
Record name | 4,4'-[(3R)-3-Hydroxyheptane-1,7-diyl]di(benzene-1,2-diol) | |
Source | EPA DSSTox | |
URL | https://comptox.epa.gov/dashboard/DTXSID80435472 | |
Description | DSSTox provides a high quality public chemistry resource for supporting improved predictive toxicology. | |
Molecular Weight |
332.4 g/mol | |
Source | PubChem | |
URL | https://pubchem.ncbi.nlm.nih.gov | |
Description | Data deposited in or computed by PubChem | |
CAS No. |
211126-61-3 | |
Record name | 4,4'-[(3R)-3-Hydroxyheptane-1,7-diyl]di(benzene-1,2-diol) | |
Source | EPA DSSTox | |
URL | https://comptox.epa.gov/dashboard/DTXSID80435472 | |
Description | DSSTox provides a high quality public chemistry resource for supporting improved predictive toxicology. | |
Retrosynthesis Analysis
AI-Powered Synthesis Planning: Our tool employs the Template_relevance Pistachio, Template_relevance Bkms_metabolic, Template_relevance Pistachio_ringbreaker, Template_relevance Reaxys, Template_relevance Reaxys_biocatalysis model, leveraging a vast database of chemical reactions to predict feasible synthetic routes.
One-Step Synthesis Focus: Specifically designed for one-step synthesis, it provides concise and direct routes for your target compounds, streamlining the synthesis process.
Accurate Predictions: Utilizing the extensive PISTACHIO, BKMS_METABOLIC, PISTACHIO_RINGBREAKER, REAXYS, REAXYS_BIOCATALYSIS database, our tool offers high-accuracy predictions, reflecting the latest in chemical research and data.
Strategy Settings
Precursor scoring | Relevance Heuristic |
---|---|
Min. plausibility | 0.01 |
Model | Template_relevance |
Template Set | Pistachio/Bkms_metabolic/Pistachio_ringbreaker/Reaxys/Reaxys_biocatalysis |
Top-N result to add to graph | 6 |
Feasible Synthetic Routes
試験管内研究製品の免責事項と情報
BenchChemで提示されるすべての記事および製品情報は、情報提供を目的としています。BenchChemで購入可能な製品は、生体外研究のために特別に設計されています。生体外研究は、ラテン語の "in glass" に由来し、生物体の外で行われる実験を指します。これらの製品は医薬品または薬として分類されておらず、FDAから任何の医療状態、病気、または疾患の予防、治療、または治癒のために承認されていません。これらの製品を人間または動物に体内に導入する形態は、法律により厳格に禁止されています。これらのガイドラインに従うことは、研究と実験において法的および倫理的な基準の遵守を確実にするために重要です。