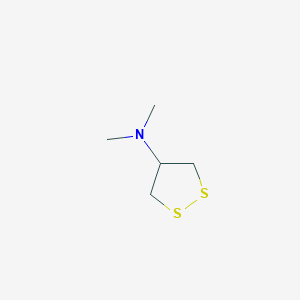
ネレイトキシン
概要
説明
Nereistoxin is a naturally occurring toxic organic compound identified as N,N-dimethyl-1,2-dithiolan-4-amine. It was first isolated in 1934 from the marine annelid Lumbriconereis heteropoda. Nereistoxin acts by blocking the nicotinic acetylcholine receptor, making it a potent neurotoxin . Researchers at Takeda Chemical Industries in Japan investigated its potential as an insecticide, leading to the development of several derivatives .
科学的研究の応用
Nereistoxin and its derivatives have a wide range of applications in scientific research:
Chemistry: Used as a model compound to study the reactivity of dithiolanes and their derivatives.
Biology: Investigated for its neurotoxic effects on various organisms, including insects and vertebrates.
Medicine: Studied for its potential use in developing new insecticides and neuroactive drugs.
Industry: Utilized in the development of insecticides for agricultural pest control.
作用機序
Target of Action
Nereistoxin’s primary target is the nicotinic acetylcholine receptor (nAChR) . This receptor plays a crucial role in transmitting signals in the nervous system. Nereistoxin acts by blocking this receptor, thereby disrupting the normal functioning of the nervous system .
Mode of Action
Nereistoxin has a chemical structure similar to acetylcholine, a neurotransmitter that binds to nAChR . It interferes with the normal functioning of nAChR, leading to a blockage of the receptor . This blockage prevents acetylcholine from binding to the receptor, thereby inhibiting the transmission of nerve impulses .
Biochemical Pathways
The primary biochemical pathway affected by nereistoxin is the cholinergic pathway . By blocking the nAChR, nereistoxin disrupts the normal flow of ions through the receptor, which is essential for the transmission of nerve impulses . This disruption affects the downstream effects of the cholinergic pathway, leading to a failure in the transmission of nerve signals.
Pharmacokinetics
It is known that nereistoxin and its synthetic analogues act as propesticides, breaking down in the environment to nereistoxin or a toxic dithiol . This suggests that nereistoxin may undergo metabolic transformations in the environment, which could potentially affect its bioavailability.
Result of Action
The result of nereistoxin’s action is a dose-dependent partial block of transmission at certain synapses in the nervous system . At higher concentrations, it can cause depolarization of the postsynaptic membrane and axonal depolarization . These effects disrupt the normal functioning of the nervous system, leading to the toxic effects of nereistoxin.
Action Environment
The action of nereistoxin can be influenced by various environmental factors. For instance, the breakdown of nereistoxin and its synthetic analogues into toxic compounds depends on environmental conditions . .
生化学分析
Biochemical Properties
Nereistoxin exhibits significant anticholinesterase activity. It has been found to inhibit human acetylcholinesterase (AChE), a key enzyme in the nervous system that breaks down acetylcholine, a neurotransmitter . This interaction with AChE is a critical aspect of Nereistoxin’s biochemical activity.
Cellular Effects
The primary cellular effect of Nereistoxin is the disruption of normal neurotransmission. By inhibiting AChE, Nereistoxin prevents the breakdown of acetylcholine in the synaptic cleft, leading to an accumulation of this neurotransmitter. This can disrupt normal cellular communication and lead to a variety of effects, including muscle paralysis in insects .
Molecular Mechanism
Nereistoxin exerts its effects at the molecular level primarily through its interaction with AChE. It binds to this enzyme and inhibits its activity, preventing it from breaking down acetylcholine. This leads to an accumulation of acetylcholine in the synaptic cleft, which can disrupt normal neurotransmission .
Temporal Effects in Laboratory Settings
It is known that Nereistoxin and its derivatives can effectively inhibit AChE activity .
Dosage Effects in Animal Models
Given its high toxicity to insects, it is likely that Nereistoxin would have significant effects at relatively low doses .
Metabolic Pathways
Given its interaction with AChE, it is likely that Nereistoxin could influence cholinergic signaling pathways .
Subcellular Localization
Given its interaction with AChE, it is likely that Nereistoxin localizes to synapses, where AChE is typically found .
準備方法
Synthetic Routes and Reaction Conditions: Nereistoxin can be synthesized through the reaction of the sodium salt of benzenethiolsulfonate with N,N-dimethyl 1,3-dichloro-2-propylamine or N,N-dimethyl 2,3-dichloropropylamine in ethanol . The reaction conditions typically involve refluxing the reactants in ethanol to yield the desired product.
Industrial Production Methods: Industrial production of nereistoxin and its derivatives involves similar synthetic routes but on a larger scale. The process includes the preparation of intermediates, purification steps, and quality control measures to ensure the consistency and purity of the final product .
化学反応の分析
Types of Reactions: Nereistoxin undergoes various chemical reactions, including:
Oxidation: Nereistoxin can be oxidized to form sulfoxides and sulfones.
Reduction: Reduction reactions can convert nereistoxin to its corresponding thiol derivatives.
Substitution: Nereistoxin can undergo nucleophilic substitution reactions, where the dimethylamino group is replaced by other nucleophiles.
Common Reagents and Conditions:
Oxidation: Common oxidizing agents include hydrogen peroxide and peracids.
Reduction: Reducing agents such as lithium aluminum hydride or sodium borohydride are used.
Substitution: Nucleophiles like thiols, amines, and halides are commonly used in substitution reactions.
Major Products:
Oxidation: Sulfoxides and sulfones.
Reduction: Thiol derivatives.
Substitution: Various substituted derivatives depending on the nucleophile used.
類似化合物との比較
Nereistoxin is unique due to its specific mode of action and chemical structure. Similar compounds include:
Bensultap: Another derivative with similar insecticidal properties.
Thiocyclam: A nereistoxin-based insecticide with a different sulfur-linked group.
Thiosultap: Another derivative with modifications to the sulfur-sulfur bond.
These compounds share the same core structure but differ in their substituents, leading to variations in their toxicity and effectiveness as insecticides .
特性
IUPAC Name |
N,N-dimethyldithiolan-4-amine | |
---|---|---|
Source | PubChem | |
URL | https://pubchem.ncbi.nlm.nih.gov | |
Description | Data deposited in or computed by PubChem | |
InChI |
InChI=1S/C5H11NS2/c1-6(2)5-3-7-8-4-5/h5H,3-4H2,1-2H3 | |
Source | PubChem | |
URL | https://pubchem.ncbi.nlm.nih.gov | |
Description | Data deposited in or computed by PubChem | |
InChI Key |
DSOOGBGKEWZRIH-UHFFFAOYSA-N | |
Source | PubChem | |
URL | https://pubchem.ncbi.nlm.nih.gov | |
Description | Data deposited in or computed by PubChem | |
Canonical SMILES |
CN(C)C1CSSC1 | |
Source | PubChem | |
URL | https://pubchem.ncbi.nlm.nih.gov | |
Description | Data deposited in or computed by PubChem | |
Molecular Formula |
C5H11NS2 | |
Source | PubChem | |
URL | https://pubchem.ncbi.nlm.nih.gov | |
Description | Data deposited in or computed by PubChem | |
Related CAS |
1631-52-3 (monooxalate), 71057-75-5 (HCl) | |
Record name | Nereistoxin | |
Source | ChemIDplus | |
URL | https://pubchem.ncbi.nlm.nih.gov/substance/?source=chemidplus&sourceid=0001631589 | |
Description | ChemIDplus is a free, web search system that provides access to the structure and nomenclature authority files used for the identification of chemical substances cited in National Library of Medicine (NLM) databases, including the TOXNET system. | |
DSSTOX Substance ID |
DTXSID0075104 | |
Record name | N,N-Dimethyl-1,2-dithiolan-4-amine | |
Source | EPA DSSTox | |
URL | https://comptox.epa.gov/dashboard/DTXSID0075104 | |
Description | DSSTox provides a high quality public chemistry resource for supporting improved predictive toxicology. | |
Molecular Weight |
149.3 g/mol | |
Source | PubChem | |
URL | https://pubchem.ncbi.nlm.nih.gov | |
Description | Data deposited in or computed by PubChem | |
CAS No. |
1631-58-9 | |
Record name | Nereistoxin | |
Source | CAS Common Chemistry | |
URL | https://commonchemistry.cas.org/detail?cas_rn=1631-58-9 | |
Description | CAS Common Chemistry is an open community resource for accessing chemical information. Nearly 500,000 chemical substances from CAS REGISTRY cover areas of community interest, including common and frequently regulated chemicals, and those relevant to high school and undergraduate chemistry classes. This chemical information, curated by our expert scientists, is provided in alignment with our mission as a division of the American Chemical Society. | |
Explanation | The data from CAS Common Chemistry is provided under a CC-BY-NC 4.0 license, unless otherwise stated. | |
Record name | Nereistoxin | |
Source | ChemIDplus | |
URL | https://pubchem.ncbi.nlm.nih.gov/substance/?source=chemidplus&sourceid=0001631589 | |
Description | ChemIDplus is a free, web search system that provides access to the structure and nomenclature authority files used for the identification of chemical substances cited in National Library of Medicine (NLM) databases, including the TOXNET system. | |
Record name | N,N-Dimethyl-1,2-dithiolan-4-amine | |
Source | EPA DSSTox | |
URL | https://comptox.epa.gov/dashboard/DTXSID0075104 | |
Description | DSSTox provides a high quality public chemistry resource for supporting improved predictive toxicology. | |
Record name | NEREISTOXIN | |
Source | FDA Global Substance Registration System (GSRS) | |
URL | https://gsrs.ncats.nih.gov/ginas/app/beta/substances/ZU7BQ8TC6K | |
Description | The FDA Global Substance Registration System (GSRS) enables the efficient and accurate exchange of information on what substances are in regulated products. Instead of relying on names, which vary across regulatory domains, countries, and regions, the GSRS knowledge base makes it possible for substances to be defined by standardized, scientific descriptions. | |
Explanation | Unless otherwise noted, the contents of the FDA website (www.fda.gov), both text and graphics, are not copyrighted. They are in the public domain and may be republished, reprinted and otherwise used freely by anyone without the need to obtain permission from FDA. Credit to the U.S. Food and Drug Administration as the source is appreciated but not required. | |
Retrosynthesis Analysis
AI-Powered Synthesis Planning: Our tool employs the Template_relevance Pistachio, Template_relevance Bkms_metabolic, Template_relevance Pistachio_ringbreaker, Template_relevance Reaxys, Template_relevance Reaxys_biocatalysis model, leveraging a vast database of chemical reactions to predict feasible synthetic routes.
One-Step Synthesis Focus: Specifically designed for one-step synthesis, it provides concise and direct routes for your target compounds, streamlining the synthesis process.
Accurate Predictions: Utilizing the extensive PISTACHIO, BKMS_METABOLIC, PISTACHIO_RINGBREAKER, REAXYS, REAXYS_BIOCATALYSIS database, our tool offers high-accuracy predictions, reflecting the latest in chemical research and data.
Strategy Settings
Precursor scoring | Relevance Heuristic |
---|---|
Min. plausibility | 0.01 |
Model | Template_relevance |
Template Set | Pistachio/Bkms_metabolic/Pistachio_ringbreaker/Reaxys/Reaxys_biocatalysis |
Top-N result to add to graph | 6 |
Feasible Synthetic Routes
Q1: What is the primary target of nereistoxin and its analogs?
A1: Nereistoxin and its analogs primarily target nicotinic acetylcholine receptors (nAChRs) in both insects and mammals. [, , , , ] These receptors are essential for synaptic transmission in the nervous system.
Q2: How does nereistoxin interact with nAChRs?
A2: Nereistoxin acts as an antagonist at nAChRs, meaning it binds to the receptor and blocks the binding of the natural neurotransmitter, acetylcholine. [, , ] This blockade disrupts nerve impulse transmission.
Q3: Does nereistoxin interact with the ionic channel of nAChRs?
A3: While nereistoxin primarily acts on the acetylcholine binding site, research suggests it may also have a minor interaction with the ionic channel of nAChRs, although this is not its primary mechanism of action. []
Q4: What are the downstream effects of nereistoxin binding to nAChRs?
A4: Blockade of nAChRs by nereistoxin leads to a variety of effects depending on the dose and the specific receptors targeted. In insects, this can result in paralysis and death. [, , , ] In mammals, nereistoxin can cause neuromuscular blockade and respiratory depression. [, ]
Q5: Is the disulfide bond in nereistoxin essential for its activity?
A5: Research suggests that the disulfide bond in nereistoxin is important for its activity. [, ] Replacing the disulfide bond with a less reactive linkage, such as in dimethylaminodithiane (DMA-DT), reduces the binding affinity for nAChRs. []
Q6: What is the molecular formula and weight of nereistoxin?
A6: The molecular formula of nereistoxin is C5H9NS2, and its molecular weight is 147.25 g/mol. [, ]
Q7: Are there any spectroscopic data available for nereistoxin?
A7: Yes, spectroscopic data, such as NMR and infrared spectroscopy, have been used to characterize nereistoxin and confirm its structure. [, , ] These techniques provide information about the molecule's functional groups and connectivity.
Q8: How do structural modifications of nereistoxin affect its activity?
A8: Modifications to the nereistoxin structure can significantly impact its activity, potency, and selectivity for different nAChR subtypes. [, , ] For example, quaternization of the amine group generally increases affinity for the Torpedo muscle and rat alpha7 nAChRs, but decreases affinity for the alpha4beta2 subtype. []
Q9: What is the effect of introducing a phosphonate group into nereistoxin analogs?
A9: Recent studies have explored incorporating phosphonate groups into nereistoxin derivatives. [] These modifications have shown promising insecticidal activity against various pests, suggesting the potential for developing new insecticides with improved properties. []
Q10: How is nereistoxin metabolized in mammals?
A10: Nereistoxin is metabolized in the liver, primarily by microsomal enzymes. [] The metabolic pathways include S-S bond cleavage, desulfuration, N-demethylation, and oxidation to sulfoxides. []
Q11: What are the main metabolites of nereistoxin?
A11: The primary metabolites of nereistoxin identified in rats include N,N-dimethyl-1,2-dithiolan-4-amine (nereistoxin), N-methyl-1,2-dithiolan-4-amine (demethylnereistoxin), 2-dimethylaminopropane, and sulfur polymers. []
Q12: What analytical methods are commonly used to detect and quantify nereistoxin and its metabolites?
A12: Various analytical techniques have been employed to detect and quantify nereistoxin and its metabolites in different matrices. These techniques include:
- Gas chromatography with flame photometric detection (GC-FPD): This method offers high sensitivity and selectivity for sulfur-containing compounds like nereistoxin. []
- Gas chromatography-mass spectrometry (GC-MS): GC-MS provides accurate identification and quantification of nereistoxin and its metabolites in complex matrices like serum, food, and animal tissues. [, , ]
- Liquid chromatography-tandem mass spectrometry (LC-MS/MS): LC-MS/MS offers high sensitivity and selectivity for analyzing nereistoxin and its metabolites in various matrices, including citrus fruits. []
- High-performance liquid chromatography with electrochemical detection (HPLC-ECD): This method allows for the simultaneous detection of both nereistoxin and dihydronereistoxin. []
Q13: How are nereistoxin residues analyzed in food samples?
A14: Nereistoxin residues in food samples are typically analyzed using methods like GC-MS or GC-MS/MS after extraction and derivatization steps. [, ] These techniques provide the sensitivity and selectivity needed to detect trace levels of nereistoxin and its metabolites in complex food matrices.
Q14: What are the potential applications of nereistoxin and its derivatives?
A15: Nereistoxin's potent insecticidal activity has led to the development of several commercial insecticides. [, , , ] Further research on nereistoxin analogs could lead to the discovery of new insecticides with improved efficacy, safety, and environmental profiles.
試験管内研究製品の免責事項と情報
BenchChemで提示されるすべての記事および製品情報は、情報提供を目的としています。BenchChemで購入可能な製品は、生体外研究のために特別に設計されています。生体外研究は、ラテン語の "in glass" に由来し、生物体の外で行われる実験を指します。これらの製品は医薬品または薬として分類されておらず、FDAから任何の医療状態、病気、または疾患の予防、治療、または治癒のために承認されていません。これらの製品を人間または動物に体内に導入する形態は、法律により厳格に禁止されています。これらのガイドラインに従うことは、研究と実験において法的および倫理的な基準の遵守を確実にするために重要です。