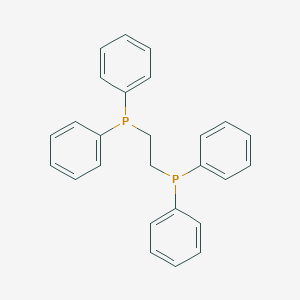
1,2-Bis(diphenylphosphino)ethane
概要
説明
1,2-Bis(diphenylphosphino)ethane (CAS: 1663-45-2), commonly abbreviated as dppe, is a bidentate diphosphine ligand with the molecular formula C₂₆H₂₄P₂ and a molecular weight of 398.42 g/mol . Structurally, it consists of two diphenylphosphine groups connected by an ethane (C₂) backbone. This ligand is a white crystalline solid with a melting point of 137–142°C and is widely used in coordination chemistry to stabilize transition metal centers, particularly in catalysis, photochemistry, and materials science . Its rigid ethane backbone and strong electron-donating phenyl groups enable the formation of stable metal complexes with well-defined geometries, making it a cornerstone in organometallic synthesis .
準備方法
Primary Synthetic Route: Alkylation of Sodium Diphenylphosphide
The most widely documented method for dppe synthesis involves the alkylation of sodium diphenylphosphide (NaPPh₂) with 1,2-dichloroethane. This reaction proceeds via nucleophilic substitution, yielding dppe and sodium chloride as a byproduct :
Reaction Scheme
2 + \text{ClCH}2\text{CH}2\text{Cl} \rightarrow \text{Ph}2\text{PCH}2\text{CH}2\text{PPh}_2 + 2 \, \text{NaCl}
Reaction Conditions and Optimization
-
Solvent Selection : Anhydrous tetrahydrofuran (THF) or diethyl ether is typically employed to maintain moisture-free conditions .
-
Temperature : Reactions are conducted at reflux (65–70°C) to ensure complete conversion .
-
Stoichiometry : A 2:1 molar ratio of NaPPh₂ to 1,2-dichloroethane minimizes side products like polymeric phosphines .
Table 1: Key Parameters for Alkylation Method
Post-reaction purification involves recrystallization from ethanol/water mixtures or benzene, yielding white crystalline dppe with melting points of 305–307°C for its dimethiodide derivative .
Alternative Methods and Derivative Synthesis
Grignard Reagent-Based Approaches
While less common, Grignard reagents have been utilized to synthesize dppe analogues. For instance, magnesium-activated aryl bromides react with diethyl phosphite to generate substituted phosphine oxides, which are subsequently reduced to phosphines :
Stepwise Process
-
Grignard Formation :
-
Phosphite Alkylation :
-
Reduction to Phosphine :
This method adapts well to sterically hindered substrates but requires stringent anhydrous conditions .
Hydrogenation of Pre-Oxidized Intermediates
Selective oxidation of dppe with hydrogen peroxide or benzyl bromide generates mono- or bis-phosphine oxides, which are hydrogenated to produce ligands like bis(dicyclohexylphosphino)ethane :
Oxidation-Hydrogenation Sequence
2\text{PCH}2\text{CH}2\text{PPh}2 \xrightarrow{\text{H}2\text{O}2} \text{Ph}2\text{P(O)CH}2\text{CH}2\text{P(O)Ph}2 \xrightarrow{\text{H}2/\text{catalyst}} \text{Cy}2\text{PCH}2\text{CH}2\text{PCy}_2
Industrial-Scale Production and Challenges
Scalability Considerations
-
Cost Efficiency : Sodium diphenylphosphide remains cost-prohibitive for large-scale synthesis, driving research into catalytic P–C bond-forming methods .
-
Byproduct Management : NaCl removal via aqueous washes and filtration is critical to prevent catalyst poisoning in downstream applications .
Table 2: Industrial vs. Laboratory-Scale Synthesis
Factor | Laboratory Scale | Industrial Scale |
---|---|---|
Reactor Volume | 0.1–1 L | 100–1000 L |
Yield | 70–80% | 85–90% (optimized) |
Purification | Recrystallization | Continuous chromatography |
Cost Drivers | Reagent purity | Solvent recovery |
Advanced Purification and Characterization
Recrystallization Protocols
-
Solvent Systems : Ethanol/water (4:1 v/v) achieves high-purity dppe, while benzene yields plate-like crystals ideal for X-ray diffraction .
-
Impurity Profiling : Residual NaCl is detected via conductivity measurements (<0.5% acceptable) .
Spectroscopic Validation
化学反応の分析
1,2-Bis(diphenylphosphino)ethane undergoes various chemical reactions, primarily due to its role as a ligand in coordination chemistry. Some of the key reactions include:
Oxidation: this compound can be oxidized to form phosphine oxides. Common oxidizing agents include hydrogen peroxide and oxygen.
Substitution: It can participate in substitution reactions where the phosphine ligands are replaced by other ligands. This is often observed in metal-catalyzed reactions.
Coordination: this compound forms stable complexes with transition metals such as palladium, platinum, and nickel.
科学的研究の応用
Ligand Properties
DPPE is known for its strong chelating ability due to its bidentate nature, which allows it to form stable five-membered chelate rings with transition metals. This property enhances the stability and reactivity of metal complexes formed with DPPE.
Table 1: Common Metal Complexes with DPPE
Metal Complex | Coordination Type | Applications |
---|---|---|
[Pd(DPPE)Cl2] | Bidentate | Catalysis in cross-coupling reactions |
[Rh(DPPE)(C2H4)] | Bidentate | Hydroformylation of alkenes |
[Ru(DPPE)(C3H5)Cl] | Bidentate | Olefin metathesis |
Case Study: Catalysis in Cross-Coupling Reactions
A study demonstrated that palladium complexes with DPPE exhibited high catalytic activity in Suzuki coupling reactions, allowing for the efficient formation of biaryl compounds from aryl halides and boronic acids .
Synthesis of Sulfides
DPPE has been utilized in a novel deoxygenation strategy that tolerates various functional groups to synthesize sulfides from alcohols. The reaction conditions allow for high yields, showcasing DPPE's versatility as a ligand .
Table 2: Reaction Conditions for Sulfide Synthesis
Reactants | Catalyst | Yield (%) | Notes |
---|---|---|---|
Alcohol + NBS | DPPE | 80-90 | Tolerates diverse functional groups |
Alcohol + PhSNa | DPPE | 75-85 | Mild reaction conditions |
Case Study: Synthesis of Thioethers
In a documented case, the use of DPPE in combination with N-bromosuccinimide (NBS) led to the successful conversion of alcohols to thioethers under mild conditions, demonstrating its effectiveness in organic synthesis .
Transition Metal Catalysis
DPPE is frequently employed in transition metal-catalyzed reactions such as hydrovinylation and alkene metathesis. Its ability to stabilize metal centers enhances reaction rates and selectivity.
Table 3: Catalytic Reactions Utilizing DPPE
Reaction Type | Metal Catalyst | Role of DPPE |
---|---|---|
Hydrovinylation | Pd | Stabilizes Pd for alkene insertion |
Alkene Metathesis | Ru | Facilitates olefin exchange |
Case Study: Hydroformylation
Research has shown that rhodium complexes with DPPE are effective catalysts for hydroformylation reactions, yielding aldehydes from alkenes with high regioselectivity .
Coordination Polymers
DPPE has been explored for the synthesis of coordination polymers, particularly those involving lanthanide metals. These polymers exhibit unique optical and electronic properties suitable for applications in photonics and sensing.
Table 4: Properties of DPPE-Based Coordination Polymers
Polymer Type | Metal Ion | Properties |
---|---|---|
Lanthanide-DPPE Complex | Eu(III) | Luminescent properties |
Lanthanide-DPPE Complex | Tb(III) | Magnetic properties |
Case Study: Luminescent Materials
A study on lanthanide complexes with DPPE revealed their potential as luminescent materials, which can be applied in display technologies and bioimaging .
作用機序
The mechanism of action of 1,2-Bis(diphenylphosphino)ethane primarily involves its role as a ligand in coordination chemistry. It forms stable complexes with transition metals, which can then participate in various catalytic processes. The phosphine ligands donate electron density to the metal center, stabilizing the metal in different oxidation states and facilitating catalytic cycles .
類似化合物との比較
Diphosphine ligands with varying backbone lengths, substituents, or donor atoms are critical for tuning the steric, electronic, and solubility properties of metal complexes. Below is a detailed comparison of dppe with structurally analogous ligands:
Backbone Length and Flexibility
Key Findings :
- The ethane backbone in dppe provides an optimal balance between rigidity and flexibility, enabling efficient chelation without excessive steric strain .
- Ligands with longer backbones (e.g., dppp) favor coordination to bulkier metals, while shorter backbones (e.g., dppm) facilitate dinuclear complexes .
Substituent Effects
Compound Name | Molecular Formula | Substituents | Electronic Effects | Steric Effects |
---|---|---|---|---|
This compound (dppe) | C₂₆H₂₄P₂ | Phenyl | Strong σ-donor; moderate π-acceptor. | High steric bulk due to phenyl groups. |
1,2-Bis(dimethylphosphino)ethane (DMPE) | C₆H₁₆P₂ | Methyl | Weaker σ-donor; no π-acceptor. | Low steric bulk, enhancing ligand accessibility. |
1,2-Bis(diethylphosphino)ethane | C₈H₂₀P₂ | Ethyl | Intermediate σ-donor strength. | Moderate steric hindrance. |
Key Findings :
- Phenyl substituents in dppe increase electron-donating ability and lipophilicity, enhancing metal complex stability but limiting solubility in polar solvents .
- Alkyl-substituted analogs (e.g., DMPE) improve solubility and reduce toxicity but exhibit weaker metal-ligand bonding, limiting catalytic activity .
Donor Atom Substitution
Compound Name | Donor Atom | Key Differences from dppe |
---|---|---|
1,2-Bis(diphenylarsino)ethane | Arsenic | Weaker σ-donor strength; larger atomic radius reduces orbital overlap with metals. |
This compound (dppe) | Phosphorus | Stronger metal-ligand bonding; preferred for high-stability complexes. |
Key Findings :
- Replacing phosphorus with arsenic decreases ligand field strength and alters redox properties, making arsenic analogs less common in catalysis but useful in niche applications like quantum information processing .
生物活性
1,2-Bis(diphenylphosphino)ethane (DPPE) is a significant compound in coordination chemistry, particularly known for its role as a bidentate ligand in metal complexes. This article explores the biological activities associated with DPPE and its derivatives, focusing on their anticancer properties, mechanisms of action, and potential therapeutic applications.
Overview of DPPE
DPPE is characterized by its ability to form stable complexes with transition metals, particularly palladium (Pd) and platinum (Pt), which are often utilized in catalysis and medicinal chemistry. Its structure allows for effective coordination to metal centers, facilitating various chemical reactions and biological interactions.
Anticancer Activity
Research indicates that DPPE and its metal complexes exhibit notable anticancer activity against various cancer cell lines. A study synthesized Pd(II) and Pt(II) complexes with DPPE and evaluated their cytotoxic effects on several cancer cell lines, including:
- MCF-7 (breast cancer)
- A549 (lung cancer)
- HCT116 (colon cancer)
- DU145 (prostate cancer)
The results showed that the cationic Pd-DPPE complex displayed significant antiproliferative activity, while neutral complexes were less effective. The active complexes induced apoptotic cell death through mechanisms involving cell cycle arrest at the S phase and increased reactive oxygen species (ROS) production, leading to mitochondrial and DNA damage .
Table 1: Cytotoxic Effects of DPPE Complexes
Complex Type | Cell Line | IC50 (µM) | Mechanism of Action |
---|---|---|---|
Neutral Pd-DPPE | MCF-7 | >100 | No significant activity |
Cationic Pd-DPPE | HCT116 | 10 | Apoptosis via ROS generation |
Neutral Pt-DPPE | A549 | >100 | No significant activity |
Cationic Pt-DPPE | DU145 | 15 | Apoptosis via cell cycle arrest |
Mechanistic Studies
Mechanistic studies have elucidated how DPPE complexes exert their biological effects. The cationic forms of these complexes interact with cellular components such as DNA and proteins, leading to:
- Cell Cycle Arrest: The complexes cause G1/S phase arrest in cancer cells.
- Apoptosis Induction: Activation of caspases and annexin V positivity indicates apoptosis.
- ROS Generation: Increased ROS levels contribute to oxidative stress, damaging cellular components .
Case Studies
Several case studies highlight the efficacy of DPPE-based metal complexes in cancer therapy:
- Pd-DPPE Complexes: A study demonstrated that these complexes showed enhanced cytotoxicity compared to cisplatin in HCT116 cells, indicating their potential as alternative chemotherapeutics .
- Pt-DPPE Complexes: Research revealed that these complexes could effectively target ovarian cancer cells, showcasing a higher antiproliferative effect compared to other phosphine ligands .
- Comparative Analysis: A comparative study on various diphosphine ligands indicated that DPPE exhibited superior biological activity against colorectal and ovarian carcinoma cell lines .
Q & A
Basic Research Questions
Q. What are the optimal synthetic routes for preparing high-purity dppe, and how can yield be improved?
dppe is typically synthesized via nucleophilic substitution between diphenylphosphine and 1,2-dibromoethane in liquid ammonia. However, yields are often low (e.g., ~0.4% in one protocol ). To improve efficiency:
- Use stoichiometric control (1:1 molar ratio of diphenylphosphine to dibromoethane) to minimize side reactions.
- Employ inert atmospheres (N₂/Ar) to prevent oxidation of phosphine intermediates.
- Purify via recrystallization from hot ethanol to achieve ≥98% purity, confirmed by melting point (138–142°C) and ¹H NMR (peaks at 1.3 ppm for CH₂, 2.3 ppm for P–CH₂, and 7.1–8.0 ppm for aromatic protons) .
Q. How is dppe characterized to confirm structural integrity in coordination chemistry?
Key characterization methods include:
- Melting point analysis : Pure dppe melts at 138–142°C; deviations suggest impurities .
- NMR spectroscopy : ¹H and ³¹P NMR identify backbone and aromatic proton environments. For example, ³¹P NMR shows a singlet at ~-15 ppm for symmetric P centers .
- X-ray crystallography : Resolves bond lengths (e.g., P–P distance ~3.2 Å) and confirms bite angle (~85° in Ru(II) complexes) .
Q. What are the most common metal complexes formed with dppe, and how do they influence reactivity?
dppe acts as a bidentate ligand, forming stable complexes with transition metals:
- Ruthenium : [Ru(dppe)₂Cl₂] adopts a trigonal-bipyramidal geometry, enabling catalytic hydrogenation .
- Nickel : [Ni(dppe)Cl₂] is used in cross-coupling reactions due to its electron-rich Ni center .
- Palladium : dppe stabilizes Pd(0) intermediates in Suzuki-Miyaura couplings, enhancing oxidative addition rates .
Advanced Research Questions
Q. How does the electronic and steric profile of dppe compare to other diphosphine ligands in catalysis?
dppe’s moderate steric bulk (cone angle ~136°) and strong σ-donor/weak π-acceptor properties differentiate it from ligands like dppm (smaller bite angle) or dppf (redox-active ferrocene backbone). Comparative studies show:
- Catalytic Activity : dppe-Pd complexes exhibit higher turnover frequencies in C–C bond formation than dppp (1,3-bis(diphenylphosphino)propane) due to optimal metal-ligand strain .
- Electronic Effects : The ethane backbone in dppe reduces π-backdonation vs. dppv (1,2-bis(diphenylphosphino)ethene), favoring electron-deficient metal centers in CO insertion reactions .
Q. What challenges arise in analyzing dppe-containing complexes using spectroscopic techniques, and how are they resolved?
- Paramagnetic Broadening : In Fe-dppe systems, paramagnetism complicates NMR. Alternative methods include:
- EPR Spectroscopy : Probes unpaired electrons in Fe(I) intermediates .
- Mössbauer Spectroscopy : Resolves oxidation states in iron clusters .
- Crystallization Issues : Air-sensitive dppe complexes require glovebox-based crystallization. Slow diffusion of hexane into THF solutions yields X-ray-quality crystals .
Q. How can contradictory data on dppe’s catalytic efficiency in hydrogenation be reconciled?
Discrepancies in literature often stem from:
- Substrate-Ligand Mismatch : Bulky substrates perform poorly with dppe due to steric constraints (e.g., <10% yield in α-pinene hydrogenation vs. >90% with dppf) .
- Solvent Effects : Polar solvents (e.g., MeOH) stabilize ionic intermediates in transfer hydrogenation, while nonpolar solvents favor neutral pathways .
Q. Methodological Considerations
Q. What safety protocols are critical when handling dppe in air-sensitive syntheses?
- Storage : Store under argon at –20°C to prevent oxidation to phosphine oxides .
- Handling : Use Schlenk lines for ligand addition to metal precursors. Monitor for exotherms during phosphine-metal binding .
- Waste Disposal : Quench residual dppe with tert-butyl hydroperoxide to oxidize toxic phosphines .
特性
IUPAC Name |
2-diphenylphosphanylethyl(diphenyl)phosphane | |
---|---|---|
Source | PubChem | |
URL | https://pubchem.ncbi.nlm.nih.gov | |
Description | Data deposited in or computed by PubChem | |
InChI |
InChI=1S/C26H24P2/c1-5-13-23(14-6-1)27(24-15-7-2-8-16-24)21-22-28(25-17-9-3-10-18-25)26-19-11-4-12-20-26/h1-20H,21-22H2 | |
Source | PubChem | |
URL | https://pubchem.ncbi.nlm.nih.gov | |
Description | Data deposited in or computed by PubChem | |
InChI Key |
QFMZQPDHXULLKC-UHFFFAOYSA-N | |
Source | PubChem | |
URL | https://pubchem.ncbi.nlm.nih.gov | |
Description | Data deposited in or computed by PubChem | |
Canonical SMILES |
C1=CC=C(C=C1)P(CCP(C2=CC=CC=C2)C3=CC=CC=C3)C4=CC=CC=C4 | |
Source | PubChem | |
URL | https://pubchem.ncbi.nlm.nih.gov | |
Description | Data deposited in or computed by PubChem | |
Molecular Formula |
C26H24P2 | |
Source | PubChem | |
URL | https://pubchem.ncbi.nlm.nih.gov | |
Description | Data deposited in or computed by PubChem | |
DSSTOX Substance ID |
DTXSID2061858 | |
Record name | Phosphine, 1,2-ethanediylbis[diphenyl- | |
Source | EPA DSSTox | |
URL | https://comptox.epa.gov/dashboard/DTXSID2061858 | |
Description | DSSTox provides a high quality public chemistry resource for supporting improved predictive toxicology. | |
Molecular Weight |
398.4 g/mol | |
Source | PubChem | |
URL | https://pubchem.ncbi.nlm.nih.gov | |
Description | Data deposited in or computed by PubChem | |
Physical Description |
White to off-white crystals; [Acros Organics MSDS] | |
Record name | Bis(1,2-diphenylphosphino)ethane | |
Source | Haz-Map, Information on Hazardous Chemicals and Occupational Diseases | |
URL | https://haz-map.com/Agents/12344 | |
Description | Haz-Map® is an occupational health database designed for health and safety professionals and for consumers seeking information about the adverse effects of workplace exposures to chemical and biological agents. | |
Explanation | Copyright (c) 2022 Haz-Map(R). All rights reserved. Unless otherwise indicated, all materials from Haz-Map are copyrighted by Haz-Map(R). No part of these materials, either text or image may be used for any purpose other than for personal use. Therefore, reproduction, modification, storage in a retrieval system or retransmission, in any form or by any means, electronic, mechanical or otherwise, for reasons other than personal use, is strictly prohibited without prior written permission. | |
CAS No. |
1663-45-2 | |
Record name | 1,2-Bis(diphenylphosphino)ethane | |
Source | CAS Common Chemistry | |
URL | https://commonchemistry.cas.org/detail?cas_rn=1663-45-2 | |
Description | CAS Common Chemistry is an open community resource for accessing chemical information. Nearly 500,000 chemical substances from CAS REGISTRY cover areas of community interest, including common and frequently regulated chemicals, and those relevant to high school and undergraduate chemistry classes. This chemical information, curated by our expert scientists, is provided in alignment with our mission as a division of the American Chemical Society. | |
Explanation | The data from CAS Common Chemistry is provided under a CC-BY-NC 4.0 license, unless otherwise stated. | |
Record name | Bis(diphenylphosphine)ethane | |
Source | ChemIDplus | |
URL | https://pubchem.ncbi.nlm.nih.gov/substance/?source=chemidplus&sourceid=0001663452 | |
Description | ChemIDplus is a free, web search system that provides access to the structure and nomenclature authority files used for the identification of chemical substances cited in National Library of Medicine (NLM) databases, including the TOXNET system. | |
Record name | 1,2-Bis(diphenylphosphino)ethane | |
Source | DTP/NCI | |
URL | https://dtp.cancer.gov/dtpstandard/servlet/dwindex?searchtype=NSC&outputformat=html&searchlist=76285 | |
Description | The NCI Development Therapeutics Program (DTP) provides services and resources to the academic and private-sector research communities worldwide to facilitate the discovery and development of new cancer therapeutic agents. | |
Explanation | Unless otherwise indicated, all text within NCI products is free of copyright and may be reused without our permission. Credit the National Cancer Institute as the source. | |
Record name | Phosphine, 1,1'-(1,2-ethanediyl)bis[1,1-diphenyl- | |
Source | EPA Chemicals under the TSCA | |
URL | https://www.epa.gov/chemicals-under-tsca | |
Description | EPA Chemicals under the Toxic Substances Control Act (TSCA) collection contains information on chemicals and their regulations under TSCA, including non-confidential content from the TSCA Chemical Substance Inventory and Chemical Data Reporting. | |
Record name | Phosphine, 1,2-ethanediylbis[diphenyl- | |
Source | EPA DSSTox | |
URL | https://comptox.epa.gov/dashboard/DTXSID2061858 | |
Description | DSSTox provides a high quality public chemistry resource for supporting improved predictive toxicology. | |
Record name | Bis(1,2-diphenylphosphino)ethane | |
Source | European Chemicals Agency (ECHA) | |
URL | https://echa.europa.eu/substance-information/-/substanceinfo/100.015.246 | |
Description | The European Chemicals Agency (ECHA) is an agency of the European Union which is the driving force among regulatory authorities in implementing the EU's groundbreaking chemicals legislation for the benefit of human health and the environment as well as for innovation and competitiveness. | |
Explanation | Use of the information, documents and data from the ECHA website is subject to the terms and conditions of this Legal Notice, and subject to other binding limitations provided for under applicable law, the information, documents and data made available on the ECHA website may be reproduced, distributed and/or used, totally or in part, for non-commercial purposes provided that ECHA is acknowledged as the source: "Source: European Chemicals Agency, http://echa.europa.eu/". Such acknowledgement must be included in each copy of the material. ECHA permits and encourages organisations and individuals to create links to the ECHA website under the following cumulative conditions: Links can only be made to webpages that provide a link to the Legal Notice page. | |
Record name | 1,2-BIS(DIPHENYLPHOSPHINO)ETHANE | |
Source | FDA Global Substance Registration System (GSRS) | |
URL | https://gsrs.ncats.nih.gov/ginas/app/beta/substances/KL33QE52I4 | |
Description | The FDA Global Substance Registration System (GSRS) enables the efficient and accurate exchange of information on what substances are in regulated products. Instead of relying on names, which vary across regulatory domains, countries, and regions, the GSRS knowledge base makes it possible for substances to be defined by standardized, scientific descriptions. | |
Explanation | Unless otherwise noted, the contents of the FDA website (www.fda.gov), both text and graphics, are not copyrighted. They are in the public domain and may be republished, reprinted and otherwise used freely by anyone without the need to obtain permission from FDA. Credit to the U.S. Food and Drug Administration as the source is appreciated but not required. | |
Retrosynthesis Analysis
AI-Powered Synthesis Planning: Our tool employs the Template_relevance Pistachio, Template_relevance Bkms_metabolic, Template_relevance Pistachio_ringbreaker, Template_relevance Reaxys, Template_relevance Reaxys_biocatalysis model, leveraging a vast database of chemical reactions to predict feasible synthetic routes.
One-Step Synthesis Focus: Specifically designed for one-step synthesis, it provides concise and direct routes for your target compounds, streamlining the synthesis process.
Accurate Predictions: Utilizing the extensive PISTACHIO, BKMS_METABOLIC, PISTACHIO_RINGBREAKER, REAXYS, REAXYS_BIOCATALYSIS database, our tool offers high-accuracy predictions, reflecting the latest in chemical research and data.
Strategy Settings
Precursor scoring | Relevance Heuristic |
---|---|
Min. plausibility | 0.01 |
Model | Template_relevance |
Template Set | Pistachio/Bkms_metabolic/Pistachio_ringbreaker/Reaxys/Reaxys_biocatalysis |
Top-N result to add to graph | 6 |
Feasible Synthetic Routes
試験管内研究製品の免責事項と情報
BenchChemで提示されるすべての記事および製品情報は、情報提供を目的としています。BenchChemで購入可能な製品は、生体外研究のために特別に設計されています。生体外研究は、ラテン語の "in glass" に由来し、生物体の外で行われる実験を指します。これらの製品は医薬品または薬として分類されておらず、FDAから任何の医療状態、病気、または疾患の予防、治療、または治癒のために承認されていません。これらの製品を人間または動物に体内に導入する形態は、法律により厳格に禁止されています。これらのガイドラインに従うことは、研究と実験において法的および倫理的な基準の遵守を確実にするために重要です。