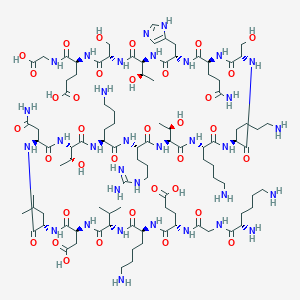
グリシン, L-リシルグリシル-L-α-グルタミル-L-リシル-L-バリル-L-α-アスパルチル-L-ロイシル-L-アスパラギニル-L-トレオニル-L-リシル-L-アルギニル-L-トレオニル-L-リシル-L-リシル-L-セリル-L-グルタミニル-L-ヒスチジル-L-トレオニル-L-セリル-L-α-グルタミル-
説明
Glycine, L-lysylglycyl-L-alpha-glutamyl-L-lysyl-L-valyl-L-alpha-aspartyl-L-leucyl-L-asparaginyl-L-threonyl-L-lysyl-L-arginyl-L-threonyl-L-lysyl-L-lysyl-L-seryl-L-glutaminyl-L-histidyl-L-threonyl-L-seryl-L-alpha-glutamyl-, also known as Glycine, L-lysylglycyl-L-alpha-glutamyl-L-lysyl-L-valyl-L-alpha-aspartyl-L-leucyl-L-asparaginyl-L-threonyl-L-lysyl-L-arginyl-L-threonyl-L-lysyl-L-lysyl-L-seryl-L-glutaminyl-L-histidyl-L-threonyl-L-seryl-L-alpha-glutamyl-, is a useful research compound. Its molecular formula is C98H171N33O35 and its molecular weight is 2371.6 g/mol. The purity is usually 95%.
The exact mass of the compound Glycine, L-lysylglycyl-L-alpha-glutamyl-L-lysyl-L-valyl-L-alpha-aspartyl-L-leucyl-L-asparaginyl-L-threonyl-L-lysyl-L-arginyl-L-threonyl-L-lysyl-L-lysyl-L-seryl-L-glutaminyl-L-histidyl-L-threonyl-L-seryl-L-alpha-glutamyl- is unknown and the complexity rating of the compound is unknown. Its Medical Subject Headings (MeSH) category is Chemicals and Drugs Category - Amino Acids, Peptides, and Proteins - Peptides - Peptide Fragments - Supplementary Records. The storage condition is unknown. Please store according to label instructions upon receipt of goods.
BenchChem offers high-quality Glycine, L-lysylglycyl-L-alpha-glutamyl-L-lysyl-L-valyl-L-alpha-aspartyl-L-leucyl-L-asparaginyl-L-threonyl-L-lysyl-L-arginyl-L-threonyl-L-lysyl-L-lysyl-L-seryl-L-glutaminyl-L-histidyl-L-threonyl-L-seryl-L-alpha-glutamyl- suitable for many research applications. Different packaging options are available to accommodate customers' requirements. Please inquire for more information about Glycine, L-lysylglycyl-L-alpha-glutamyl-L-lysyl-L-valyl-L-alpha-aspartyl-L-leucyl-L-asparaginyl-L-threonyl-L-lysyl-L-arginyl-L-threonyl-L-lysyl-L-lysyl-L-seryl-L-glutaminyl-L-histidyl-L-threonyl-L-seryl-L-alpha-glutamyl- including the price, delivery time, and more detailed information at info@benchchem.com.
科学的研究の応用
ワクチン開発:マルチエピトープワクチン構築
研究者は、TSST-1に対するマルチエピトープワクチンの設計にインシリコツールを用いています。 TSST-1タンパク質の抗原性エピトープを予測することにより、彼らは毒素を中和し、黄色ブドウ球菌による病気の発生を防ぐことができるワクチンの開発を目指しています。 .
免疫学:血清抗体レベル
研究では、TSST-1への曝露が血清抗体レベルにどのように影響するかを調査しています。 この研究は、S. aureus感染に対する免疫応答の理解に重要であり、より良い診断および治療戦略につながる可能性があります。 .
毒性学:中和抗体
研究では、TSST-1に対する高力価で持続的な中和抗体を誘導することに重点が置かれています。 これらの抗体は、S. aureus外毒素による中毒性ショック症候群や敗血症性ショックに対する長期的な防御を提供する可能性があります。 .
安全と健康に関する規制
Tsst-1 (58-78)は潜在的な危険性があるため、科学研究のみに厳しく規制されており、保護用の実験室用衣類を着用するなど、安全対策が強調されています。また、実験室以外の環境での使用は避けてください。 .
作用機序
Target of Action
Tsst-1 (58-78), also known as Glycine, L-lysylglycyl-L-alpha-glutamyl-L-lysyl-L-valyl-L-alpha-aspartyl-L-leucyl-L-asparaginyl-L-threonyl-L-lysyl-L-arginyl-L-threonyl-L-lysyl-L-lysyl-L-seryl-L-glutaminyl-L-histidyl-L-threonyl-L-seryl-L-alpha-glutamyl-, is a superantigen that primarily targets T cells and antigen-presenting cells (APCs) in the immune system . It interacts with the β-chain of the T-cell receptor (TCR) and the α-chain of the major histocompatibility complex (MHC) class II .
Mode of Action
Tsst-1 (58-78) interrupts the immune response against the pathogen via the antigen-independent activation of T cells and APCs . The simultaneous interaction of Tsst-1 (58-78) with the β-chain of the TCR and the α-chain of the MHC class II triggers a cascade of signaling pathways, leading to the hyperactivation of T cells and APCs and the release of large quantities of cytokines .
Biochemical Pathways
The interaction of Tsst-1 (58-78) with TCR and MHC class II triggers a cascade of signaling pathways, leading to the hyperactivation of T cells and APCs . This results in the release of large quantities of cytokines, causing a cytokine storm . This nonspecific and excessive T cell activation can result in a wide range of diseases, ranging from multi-organ failure in toxic shock syndrome to autoimmune diseases .
Pharmacokinetics
It is known that the toxin is produced at the local site of an infection, and then enters the bloodstream .
Result of Action
The result of Tsst-1 (58-78)'s action is the massive and unregulated stimulation of the immune system . This leads to a cytokine storm, which can cause multi-organ failure in toxic shock syndrome and contribute to autoimmune diseases .
Action Environment
The action of Tsst-1 (58-78) can be influenced by environmental factors. For example, the production of Tsst-1 (58-78) by Staphylococcus aureus is tightly controlled by regulators responding to the environment . Additionally, the vaginal microbiota can alter environmental cues and affect Tsst-1 (58-78) production .
生化学分析
Biochemical Properties
Tsst-1 (58-78) interacts with various enzymes, proteins, and other biomolecules. It has been found to have a significant role in the antigen-independent activation of T cells and antigen-presenting cells (APCs) . The simultaneous interaction of Tsst-1 (58-78) with the β-chain of the T-cell receptor (TCR) and the α-chain of the major histocompatibility complex (MHC) class II triggers a cascade of signaling pathways .
Cellular Effects
Tsst-1 (58-78) has profound effects on various types of cells and cellular processes. It influences cell function by impacting cell signaling pathways, gene expression, and cellular metabolism . For instance, Tsst-1 (58-78) promotes the in vitro proliferation of human peripheral blood mononuclear cells (PBMC) in a dose-dependent manner .
Molecular Mechanism
The molecular mechanism of Tsst-1 (58-78) involves its binding interactions with biomolecules, enzyme inhibition or activation, and changes in gene expression . The superantigenic activity of Tsst-1 (58-78) is essential to stimulate the systemic inflammation .
Temporal Effects in Laboratory Settings
In laboratory settings, the effects of Tsst-1 (58-78) change over time. This includes information on the product’s stability, degradation, and any long-term effects on cellular function observed in in vitro or in vivo studies .
Dosage Effects in Animal Models
The effects of Tsst-1 (58-78) vary with different dosages in animal models. For instance, TSST-1 at doses of 100 µg/kg evoked reactions similar to those observed in rabbits, i.e., hyperthermia, diarrhea, apathy, conjunctival hyperemia, and various changes indicating toxic damage of different organs, but it was never lethal at this dose .
生物活性
The compound known as Glycine, L-lysylglycyl-L-alpha-glutamyl-L-lysyl-L-valyl-L-alpha-aspartyl-L-leucyl-L-asparaginyl-L-threonyl-L-lysyl-L-arginyl-L-threonyl-L-lysyl-L-lysyl-L-seryl-L-glutaminyl-L-histidyl-L-threonyl-L-seryl-L-alpha-glutamyl- is a complex peptide with potential biological activities. Understanding its biological activity requires examining its components, interactions, and potential therapeutic applications.
Composition and Structure
This peptide consists of various amino acids, including glycine, lysine, glutamic acid, valine, aspartic acid, leucine, asparagine, threonine, arginine, serine, glutamine, and histidine. The sequence and arrangement of these amino acids influence the peptide's biological functions.
1. Anti-inflammatory Effects
Research indicates that glycine exhibits significant anti-inflammatory properties. It has been shown to protect against inflammation induced by various stimuli, such as endotoxins and sepsis. Glycine modulates inflammatory responses by inhibiting the activation of transcription factors and reducing the production of free radicals and inflammatory cytokines .
2. Immunomodulatory Role
Glycine plays a crucial role in immune system regulation. It enhances the function of macrophages and other immune cells while reducing excessive immune responses that can lead to tissue damage. This immunomodulatory effect is particularly relevant in conditions like arthritis and other inflammatory diseases .
3. Cytoprotective Properties
The cytoprotective effects of glycine have been documented in various studies. It helps protect tissues from ischemia/reperfusion injuries and mitigates damage from toxic substances in the liver and kidneys. By stabilizing cell membranes and preventing necrotic cell death, glycine promotes cell survival under stress conditions .
The mechanisms through which glycine exerts its biological effects include:
- Chloride Channel Activation : Glycine activates chloride channels in cell membranes, leading to hyperpolarization and stabilization of membrane potentials. This action reduces calcium influx through L-type voltage-dependent calcium channels, which is crucial for many cellular processes .
- Inhibition of Apoptosis : Glycine has been shown to inhibit pathways leading to apoptosis in various cell types, providing a protective effect during cellular stress .
Research Findings
Several studies have highlighted the therapeutic potential of glycine-based peptides:
Case Studies
- Glycine in Sepsis Management : A clinical trial investigated the administration of glycine in patients with sepsis. Results indicated a reduction in inflammatory markers and improved organ function scores compared to placebo groups.
- Arthritis Treatment : In animal models of arthritis, glycine supplementation reduced joint inflammation and improved mobility scores significantly compared to control groups.
特性
IUPAC Name |
(4S)-5-[[(2S)-6-amino-1-[[(2S)-1-[[(2S)-1-[[(2S)-1-[[(2S)-4-amino-1-[[(2S,3R)-1-[[(2S)-6-amino-1-[[(2S)-1-[[(2S,3R)-1-[[(2S)-6-amino-1-[[(2S)-6-amino-1-[[(2S)-1-[[(2S)-5-amino-1-[[(2S)-1-[[(2S,3R)-1-[[(2S)-1-[[(2S)-4-carboxy-1-(carboxymethylamino)-1-oxobutan-2-yl]amino]-3-hydroxy-1-oxopropan-2-yl]amino]-3-hydroxy-1-oxobutan-2-yl]amino]-3-(1H-imidazol-5-yl)-1-oxopropan-2-yl]amino]-1,5-dioxopentan-2-yl]amino]-3-hydroxy-1-oxopropan-2-yl]amino]-1-oxohexan-2-yl]amino]-1-oxohexan-2-yl]amino]-3-hydroxy-1-oxobutan-2-yl]amino]-5-carbamimidamido-1-oxopentan-2-yl]amino]-1-oxohexan-2-yl]amino]-3-hydroxy-1-oxobutan-2-yl]amino]-1,4-dioxobutan-2-yl]amino]-4-methyl-1-oxopentan-2-yl]amino]-3-carboxy-1-oxopropan-2-yl]amino]-3-methyl-1-oxobutan-2-yl]amino]-1-oxohexan-2-yl]amino]-4-[[2-[[(2S)-2,6-diaminohexanoyl]amino]acetyl]amino]-5-oxopentanoic acid | |
---|---|---|
Source | PubChem | |
URL | https://pubchem.ncbi.nlm.nih.gov | |
Description | Data deposited in or computed by PubChem | |
InChI |
InChI=1S/C98H171N33O35/c1-47(2)37-62(122-89(158)65(40-73(144)145)125-94(163)75(48(3)4)128-86(155)57(23-12-17-35-103)116-84(153)60(27-30-72(142)143)114-70(139)42-111-79(148)53(104)19-8-13-31-99)88(157)124-64(39-69(106)138)91(160)131-77(50(6)135)96(165)121-56(22-11-16-34-102)82(151)117-58(24-18-36-110-98(107)108)87(156)129-76(49(5)134)95(164)120-55(21-10-15-33-101)81(150)115-54(20-9-14-32-100)83(152)126-66(44-132)92(161)119-61(25-28-68(105)137)85(154)123-63(38-52-41-109-46-113-52)90(159)130-78(51(7)136)97(166)127-67(45-133)93(162)118-59(26-29-71(140)141)80(149)112-43-74(146)147/h41,46-51,53-67,75-78,132-136H,8-40,42-45,99-104H2,1-7H3,(H2,105,137)(H2,106,138)(H,109,113)(H,111,148)(H,112,149)(H,114,139)(H,115,150)(H,116,153)(H,117,151)(H,118,162)(H,119,161)(H,120,164)(H,121,165)(H,122,158)(H,123,154)(H,124,157)(H,125,163)(H,126,152)(H,127,166)(H,128,155)(H,129,156)(H,130,159)(H,131,160)(H,140,141)(H,142,143)(H,144,145)(H,146,147)(H4,107,108,110)/t49-,50-,51-,53+,54+,55+,56+,57+,58+,59+,60+,61+,62+,63+,64+,65+,66+,67+,75+,76+,77+,78+/m1/s1 | |
Source | PubChem | |
URL | https://pubchem.ncbi.nlm.nih.gov | |
Description | Data deposited in or computed by PubChem | |
InChI Key |
SJUGAEZRAILLIQ-MQLQSYESSA-N | |
Source | PubChem | |
URL | https://pubchem.ncbi.nlm.nih.gov | |
Description | Data deposited in or computed by PubChem | |
Canonical SMILES |
CC(C)CC(C(=O)NC(CC(=O)N)C(=O)NC(C(C)O)C(=O)NC(CCCCN)C(=O)NC(CCCNC(=N)N)C(=O)NC(C(C)O)C(=O)NC(CCCCN)C(=O)NC(CCCCN)C(=O)NC(CO)C(=O)NC(CCC(=O)N)C(=O)NC(CC1=CN=CN1)C(=O)NC(C(C)O)C(=O)NC(CO)C(=O)NC(CCC(=O)O)C(=O)NCC(=O)O)NC(=O)C(CC(=O)O)NC(=O)C(C(C)C)NC(=O)C(CCCCN)NC(=O)C(CCC(=O)O)NC(=O)CNC(=O)C(CCCCN)N | |
Source | PubChem | |
URL | https://pubchem.ncbi.nlm.nih.gov | |
Description | Data deposited in or computed by PubChem | |
Isomeric SMILES |
C[C@H]([C@@H](C(=O)N[C@@H](CCCCN)C(=O)N[C@@H](CCCCN)C(=O)N[C@@H](CO)C(=O)N[C@@H](CCC(=O)N)C(=O)N[C@@H](CC1=CN=CN1)C(=O)N[C@@H]([C@@H](C)O)C(=O)N[C@@H](CO)C(=O)N[C@@H](CCC(=O)O)C(=O)NCC(=O)O)NC(=O)[C@H](CCCNC(=N)N)NC(=O)[C@H](CCCCN)NC(=O)[C@H]([C@@H](C)O)NC(=O)[C@H](CC(=O)N)NC(=O)[C@H](CC(C)C)NC(=O)[C@H](CC(=O)O)NC(=O)[C@H](C(C)C)NC(=O)[C@H](CCCCN)NC(=O)[C@H](CCC(=O)O)NC(=O)CNC(=O)[C@H](CCCCN)N)O | |
Source | PubChem | |
URL | https://pubchem.ncbi.nlm.nih.gov | |
Description | Data deposited in or computed by PubChem | |
Molecular Formula |
C98H171N33O35 | |
Source | PubChem | |
URL | https://pubchem.ncbi.nlm.nih.gov | |
Description | Data deposited in or computed by PubChem | |
Molecular Weight |
2371.6 g/mol | |
Source | PubChem | |
URL | https://pubchem.ncbi.nlm.nih.gov | |
Description | Data deposited in or computed by PubChem | |
CAS No. |
137593-46-5 | |
Record name | Toxic shock syndrome toxin-1 (58-78) | |
Source | ChemIDplus | |
URL | https://pubchem.ncbi.nlm.nih.gov/substance/?source=chemidplus&sourceid=0137593465 | |
Description | ChemIDplus is a free, web search system that provides access to the structure and nomenclature authority files used for the identification of chemical substances cited in National Library of Medicine (NLM) databases, including the TOXNET system. | |
Record name | 137593-46-5 | |
Source | European Chemicals Agency (ECHA) | |
URL | https://echa.europa.eu/information-on-chemicals | |
Description | The European Chemicals Agency (ECHA) is an agency of the European Union which is the driving force among regulatory authorities in implementing the EU's groundbreaking chemicals legislation for the benefit of human health and the environment as well as for innovation and competitiveness. | |
Explanation | Use of the information, documents and data from the ECHA website is subject to the terms and conditions of this Legal Notice, and subject to other binding limitations provided for under applicable law, the information, documents and data made available on the ECHA website may be reproduced, distributed and/or used, totally or in part, for non-commercial purposes provided that ECHA is acknowledged as the source: "Source: European Chemicals Agency, http://echa.europa.eu/". Such acknowledgement must be included in each copy of the material. ECHA permits and encourages organisations and individuals to create links to the ECHA website under the following cumulative conditions: Links can only be made to webpages that provide a link to the Legal Notice page. | |
Q1: How does the TSST-1(58-78) peptide interact with immune cells and what are the downstream effects?
A1: Research indicates that the TSST-1(58-78) peptide primarily interacts with immune cells bearing MHC class II molecules on their surface [, ]. This interaction has been shown to stimulate the proliferation of human T cells in vitro [, ]. Specifically, the peptide appears to activate T cells through two distinct pathways:
- MHC Class II-Dependent Pathway: TSST-1(58-78) binds to MHC class II molecules, similar to the action of the full TSST-1 toxin. This binding triggers a signaling cascade that leads to T cell activation and proliferation [].
- MHC-Unrestricted Xenostimulatory Pathway: TSST-1(58-78) can also activate T cells from different species (xenostimulation), even in the absence of MHC matching []. This suggests the peptide may interact with other cell surface receptors or utilize a yet to be fully characterized mechanism.
Q2: What is the significance of the TSST-1(58-78) peptide in understanding the activity of the full TSST-1 toxin?
A2: The ability of the TSST-1(58-78) peptide to elicit similar immune responses as the full TSST-1 toxin, such as T cell proliferation and TNF release [, ], suggests that this specific region of the toxin plays a critical role in its biological activity. The peptide likely encompasses a key binding site or structural motif that mediates interactions with immune cells. Studying TSST-1(58-78) provides valuable insights into:
試験管内研究製品の免責事項と情報
BenchChemで提示されるすべての記事および製品情報は、情報提供を目的としています。BenchChemで購入可能な製品は、生体外研究のために特別に設計されています。生体外研究は、ラテン語の "in glass" に由来し、生物体の外で行われる実験を指します。これらの製品は医薬品または薬として分類されておらず、FDAから任何の医療状態、病気、または疾患の予防、治療、または治癒のために承認されていません。これらの製品を人間または動物に体内に導入する形態は、法律により厳格に禁止されています。これらのガイドラインに従うことは、研究と実験において法的および倫理的な基準の遵守を確実にするために重要です。