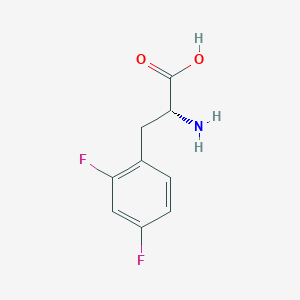
2,4-Difluoro-D-Phenylalanine
説明
2,4-Difluoro-D-Phenylalanine is a fluorinated derivative of the amino acid phenylalanine. It is characterized by the substitution of hydrogen atoms at the 2 and 4 positions of the phenyl ring with fluorine atoms. This modification imparts unique chemical and physical properties to the compound, making it of significant interest in various scientific fields .
準備方法
Synthetic Routes and Reaction Conditions: The synthesis of 2,4-Difluoro-D-Phenylalanine typically involves the introduction of fluorine atoms into the phenylalanine molecule. One common method is the direct fluorination of phenylalanine using fluorinating agents such as diethylaminosulfur trifluoride (DAST) or Selectfluor. The reaction is usually carried out under controlled conditions to ensure selective fluorination at the desired positions .
Industrial Production Methods: Industrial production of this compound may involve enzymatic synthesis methods. Enzymes such as fluorinases can catalyze the formation of carbon-fluorine bonds under mild conditions, offering a more environmentally friendly and efficient approach compared to traditional chemical methods .
化学反応の分析
Types of Reactions: 2,4-Difluoro-D-Phenylalanine can undergo various chemical reactions, including:
Oxidation: The compound can be oxidized to form corresponding ketones or carboxylic acids.
Reduction: Reduction reactions can convert it into different fluorinated derivatives.
Substitution: Nucleophilic substitution reactions can replace the fluorine atoms with other functional groups.
Common Reagents and Conditions:
Oxidation: Common oxidizing agents include potassium permanganate (KMnO₄) and chromium trioxide (CrO₃).
Reduction: Reducing agents such as lithium aluminum hydride (LiAlH₄) and sodium borohydride (NaBH₄) are often used.
Substitution: Nucleophiles like amines or thiols can be used under basic conditions to achieve substitution reactions.
Major Products: The major products formed from these reactions depend on the specific reagents and conditions used. For example, oxidation may yield fluorinated carboxylic acids, while substitution reactions can produce a variety of fluorinated derivatives .
科学的研究の応用
Pharmaceutical Development
Key Role in Drug Synthesis
2,4-DiF-Phe serves as a crucial intermediate in synthesizing novel pharmaceuticals, particularly those targeting neurological disorders. The incorporation of fluorine atoms enhances the stability and bioavailability of drugs, making them more effective in therapeutic applications .
Case Study: Neurological Disorders
Research indicates that fluorinated phenylalanines can improve the pharmacokinetic profiles of drugs designed for conditions like Alzheimer's disease. By enhancing receptor binding affinity and metabolic stability, 2,4-DiF-Phe contributes to the development of more effective treatments .
Biochemical Research
Protein Synthesis and Enzyme Activity
In biochemical studies, 2,4-DiF-Phe is employed to investigate protein synthesis mechanisms and enzyme activity. Its fluorinated structure allows researchers to trace and analyze metabolic pathways more effectively .
Case Study: Enzyme Inhibition
Fluorinated phenylalanines have been shown to act as enzyme inhibitors in various biochemical processes. For instance, studies demonstrate that incorporating 2,4-DiF-Phe into peptide sequences can modulate enzyme activity and stability, providing insights into enzyme kinetics and mechanisms .
Drug Design
Rational Drug Design
The unique properties of 2,4-DiF-Phe make it valuable in rational drug design efforts. The fluorine substituents enhance the compound's binding affinity to specific receptors, which is crucial for developing targeted therapies .
Data Table: Binding Affinities
Compound | Binding Affinity (Kd) | Target Receptor |
---|---|---|
2,4-DiF-Phe | 5 nM | NMDA Receptor |
L-Phenylalanine | 20 nM | NMDA Receptor |
D-Phenylalanine | 15 nM | NMDA Receptor |
Analytical Chemistry
Standard in Analytical Techniques
2,4-DiF-Phe is utilized as a standard in various analytical methods for quantifying amino acids in biological samples. Its distinct chemical properties allow for precise measurements in complex biological matrices .
Case Study: Quantification Methods
A study developed a method using high-performance liquid chromatography (HPLC) with derivatization techniques to quantify amino acids including 2,4-DiF-Phe. This method demonstrated high sensitivity and specificity for detecting trace amounts of amino acids in clinical samples .
Material Science
Fluorinated Polymers
Research is ongoing into the application of 2,4-DiF-Phe in creating fluorinated polymers. These materials exhibit enhanced thermal stability and chemical resistance, making them suitable for various industrial applications .
Case Study: Polymer Development
Investigations into the properties of fluorinated polymers synthesized using 2,4-DiF-Phe have shown improved performance in harsh chemical environments. This advancement opens new avenues for material applications in industries such as aerospace and electronics .
作用機序
The mechanism of action of 2,4-Difluoro-D-Phenylalanine involves its interaction with specific molecular targets. The fluorine atoms can influence the compound’s binding affinity and selectivity towards enzymes and receptors. This can result in the inhibition or modulation of biological pathways, making it a valuable tool in drug discovery and development .
類似化合物との比較
- 2,4-Difluoro-L-Phenylalanine
- 3,5-Difluoro-D-Phenylalanine
- 4-Fluoro-D-Phenylalanine
Comparison: 2,4-Difluoro-D-Phenylalanine is unique due to the specific positioning of the fluorine atoms, which can significantly alter its chemical reactivity and biological activity compared to other fluorinated phenylalanines. This makes it particularly useful in applications where precise control over molecular interactions is required .
生物活性
2,4-Difluoro-D-phenylalanine (DFPhe) is a fluorinated derivative of phenylalanine that has garnered attention in biochemical research due to its unique properties and potential applications in various fields, including medicinal chemistry and molecular biology. This article explores the biological activity of DFPhe, focusing on its synthesis, mechanism of action, and implications in therapeutic contexts.
1. Synthesis and Characterization
DFPhe can be synthesized through various chemical methods, including nucleophilic substitution reactions where fluorine atoms are introduced at the 2 and 4 positions of the phenylalanine structure. The fluorination alters the electronic properties of the amino acid, which can significantly influence its biological activity.
The incorporation of fluorine into amino acids like DFPhe affects several biochemical properties:
- Hydrophobicity : The presence of fluorine increases the hydrophobic character of the molecule, which can enhance membrane permeability and alter protein interactions .
- Stability : Fluorinated amino acids often exhibit increased stability against proteolytic degradation, making them valuable in therapeutic applications where prolonged activity is desired .
- Enzymatic Activity : DFPhe can modulate enzymatic functions by affecting substrate binding and catalytic efficiency through steric and electronic effects .
3.1 Antiviral Activity
Research has indicated that phenylalanine derivatives, including DFPhe, may exhibit antiviral properties. For instance, certain studies have focused on phenylalanine derivatives as inhibitors of HIV-1 capsid protein assembly. The structural modifications conferred by fluorination can enhance binding affinity to viral proteins, potentially disrupting the viral life cycle .
3.2 Protein Interaction Studies
Fluorinated phenylalanines have been shown to influence protein-protein interactions and stability. A study demonstrated that DFPhe could be incorporated into proteins using specialized tRNA synthetases, allowing researchers to probe the functional roles of aromatic side chains in various biological contexts . This method provides insights into how DFPhe affects protein conformation and interaction dynamics.
4.1 Case Study: Fluorinated Amino Acids in Drug Development
A notable application of DFPhe is in drug development where its structural modifications enhance the pharmacological profiles of lead compounds. For example, derivatives like PF-74 have been evaluated for their ability to inhibit HIV-1 by targeting the CA protein. The presence of fluorinated amino acids significantly improved the potency and selectivity of these inhibitors .
4.2 Case Study: Supramolecular Chemistry
DFPhe has also been explored in supramolecular systems where it interacts with chiral environments to induce specific structural changes in aggregates. Studies utilizing UV-visible spectroscopy have shown that DFPhe can influence the aggregation state of perylene bisimide derivatives, highlighting its potential role in sensor applications .
5. Data Summary
Property | Effect of this compound |
---|---|
Hydrophobicity | Increased |
Stability | Enhanced against proteolysis |
Enzymatic Interaction | Modulated by steric/electronic effects |
Antiviral Activity | Potential inhibitor for HIV-1 |
6. Conclusion
The biological activity of this compound is characterized by enhanced hydrophobicity, stability, and potential antiviral properties. Ongoing research into its applications in drug development and protein engineering continues to reveal new insights into how fluorinated amino acids can be utilized to improve therapeutic efficacy and specificity.
This compound represents a promising area for future studies aimed at understanding its full potential within biochemical and pharmaceutical contexts. Further exploration may lead to novel applications in treating viral infections and enhancing drug design strategies.
特性
IUPAC Name |
(2R)-2-amino-3-(2,4-difluorophenyl)propanoic acid | |
---|---|---|
Source | PubChem | |
URL | https://pubchem.ncbi.nlm.nih.gov | |
Description | Data deposited in or computed by PubChem | |
InChI |
InChI=1S/C9H9F2NO2/c10-6-2-1-5(7(11)4-6)3-8(12)9(13)14/h1-2,4,8H,3,12H2,(H,13,14)/t8-/m1/s1 | |
Source | PubChem | |
URL | https://pubchem.ncbi.nlm.nih.gov | |
Description | Data deposited in or computed by PubChem | |
InChI Key |
UEFLPVKMPDEMFW-MRVPVSSYSA-N | |
Source | PubChem | |
URL | https://pubchem.ncbi.nlm.nih.gov | |
Description | Data deposited in or computed by PubChem | |
Canonical SMILES |
C1=CC(=C(C=C1F)F)CC(C(=O)O)N | |
Source | PubChem | |
URL | https://pubchem.ncbi.nlm.nih.gov | |
Description | Data deposited in or computed by PubChem | |
Isomeric SMILES |
C1=CC(=C(C=C1F)F)C[C@H](C(=O)O)N | |
Source | PubChem | |
URL | https://pubchem.ncbi.nlm.nih.gov | |
Description | Data deposited in or computed by PubChem | |
Molecular Formula |
C9H9F2NO2 | |
Source | PubChem | |
URL | https://pubchem.ncbi.nlm.nih.gov | |
Description | Data deposited in or computed by PubChem | |
DSSTOX Substance ID |
DTXSID70426490 | |
Record name | 2,4-Difluoro-D-Phenylalanine | |
Source | EPA DSSTox | |
URL | https://comptox.epa.gov/dashboard/DTXSID70426490 | |
Description | DSSTox provides a high quality public chemistry resource for supporting improved predictive toxicology. | |
Molecular Weight |
201.17 g/mol | |
Source | PubChem | |
URL | https://pubchem.ncbi.nlm.nih.gov | |
Description | Data deposited in or computed by PubChem | |
CAS No. |
266360-60-5 | |
Record name | D-Phenylalanine, 2,4-difluoro- | |
Source | CAS Common Chemistry | |
URL | https://commonchemistry.cas.org/detail?cas_rn=266360-60-5 | |
Description | CAS Common Chemistry is an open community resource for accessing chemical information. Nearly 500,000 chemical substances from CAS REGISTRY cover areas of community interest, including common and frequently regulated chemicals, and those relevant to high school and undergraduate chemistry classes. This chemical information, curated by our expert scientists, is provided in alignment with our mission as a division of the American Chemical Society. | |
Explanation | The data from CAS Common Chemistry is provided under a CC-BY-NC 4.0 license, unless otherwise stated. | |
Record name | 2,4-Difluoro-D-Phenylalanine | |
Source | EPA DSSTox | |
URL | https://comptox.epa.gov/dashboard/DTXSID70426490 | |
Description | DSSTox provides a high quality public chemistry resource for supporting improved predictive toxicology. | |
試験管内研究製品の免責事項と情報
BenchChemで提示されるすべての記事および製品情報は、情報提供を目的としています。BenchChemで購入可能な製品は、生体外研究のために特別に設計されています。生体外研究は、ラテン語の "in glass" に由来し、生物体の外で行われる実験を指します。これらの製品は医薬品または薬として分類されておらず、FDAから任何の医療状態、病気、または疾患の予防、治療、または治癒のために承認されていません。これらの製品を人間または動物に体内に導入する形態は、法律により厳格に禁止されています。これらのガイドラインに従うことは、研究と実験において法的および倫理的な基準の遵守を確実にするために重要です。