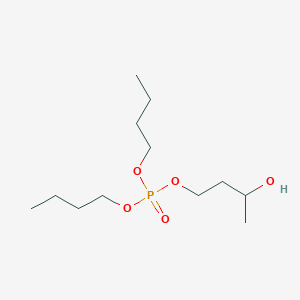
ジブチル3-ヒドロキシブチルリン酸
概要
説明
Dibutyl-3-Hydroxybutyl Phosphate is an organophosphorus compound produced from the metabolism of tributyl phosphate. It is known for its role in various biochemical and industrial processes. The compound has the molecular formula C12H27O5P and a molecular weight of 282.3 g/mol .
科学的研究の応用
Chemical Properties and Structure
DBHBP has the molecular formula and a molecular weight of 282.3 g/mol. It is characterized by its high purity, typically exceeding 95% in research settings. The compound exists primarily in solution form, often in methanol, and exhibits biological activity related to its role as a metabolite of TBP.
Biochemical Research Applications
DBHBP is utilized in various biochemical research contexts, including:
- Metabolic Studies : As a metabolite of TBP, DBHBP plays a role in studies examining phosphoric acid metabolism and enzyme interactions. Its effects on cellular processes are under investigation, particularly concerning phospholipid membrane interactions .
- Reference Compound : DBHBP is employed as a reference compound in biochemical assays, helping to elucidate the metabolic pathways involving organophosphates.
- Interaction Studies : Research focuses on DBHBP's interactions with enzymes and cellular components, providing insights into its biological activities and potential therapeutic applications.
Toxicological Evaluations
Toxicological assessments have highlighted several important findings regarding DBHBP:
- Metabolism and Excretion : Studies indicate that DBHBP is formed during the metabolism of TBP, with significant urinary metabolites identified. For instance, dibutyl phosphate is a major urinary metabolite following TBP exposure .
- Acute Toxicity : DBHBP is classified as having acute toxicity (Category 4), with median lethal doses (LD50) ranging from 1164 to 3350 mg/kg in rats. This classification underscores the need for careful handling and usage in research settings .
- Chronic Exposure Effects : Long-term studies on TBP have shown dose-dependent changes in urinary bladder epithelium and other histopathological findings. These studies provide context for understanding the potential impacts of DBHBP as a metabolite of TBP .
Environmental Applications
DBHBP's role extends into environmental science, particularly concerning:
- Biodegradation Studies : Research has been conducted on the degradation of organophosphate flame retardants by white-rot fungi, where DBHBP was identified as one of the metabolites. This highlights its potential significance in bioremediation efforts .
- Ecotoxicology : The environmental fate of TBP and its metabolites, including DBHBP, is crucial for assessing ecological risks associated with their industrial use. Understanding their degradation pathways can inform safety regulations and environmental protection measures .
作用機序
ジブチル-3-ヒドロキシブチルホスフェートの作用機序には、様々な分子標的および経路との相互作用が含まれます。有機リン化合物の代謝に影響を与え、生物系における酵素活性を変化させることが知られています。この化合物の効果は、特定の酵素や受容体との相互作用を介して媒介され、細胞プロセスに変化をもたらします .
類似化合物:
トリブチルホスフェート: ジブチル-3-ヒドロキシブチルホスフェートの前駆体であり、同様の工業用途で使用されています。
ジブチルホスフェート: トリブチルホスフェートの別の代謝物であり、同様の特性を持っています。
リン酸エステル: 類似の化学構造と反応性を有するより広範な化合物のクラスです。
ユニークさ: ジブチル-3-ヒドロキシブチルホスフェートは、その特異的な代謝起源とその独特の化学的性質のためにユニークです。様々な化学反応を起こす能力と、様々な分野における幅広い用途は、研究と工業利用にとって貴重な化合物となっています .
準備方法
Synthetic Routes and Reaction Conditions: Dibutyl-3-Hydroxybutyl Phosphate can be synthesized through the metabolism of tributyl phosphate. This process involves the incubation of tributyl phosphate with goldfish liver microsomes in the presence of NADPH, resulting in the formation of dibutyl-3-hydroxybutyl phosphate and dibutyl phosphate .
Industrial Production Methods: Industrial production of Dibutyl-3-Hydroxybutyl Phosphate typically involves the radiolysis of tributyl phosphate. This method has been shown to produce the compound efficiently .
化学反応の分析
反応の種類: ジブチル-3-ヒドロキシブチルホスフェートは、酸化、還元、置換反応などの様々な化学反応を起こします。
一般的な試薬と条件:
酸化: この化合物は、過マンガン酸カリウムや三酸化クロムなどの強力な酸化剤を用いて酸化できます。
還元: 還元は、水素化リチウムアルミニウムなどの還元剤を用いて行うことができます。
置換: 置換反応は、しばしば水酸化物イオンやアミンなどの求核剤を伴います。
生成される主な生成物: これらの反応から生成される主な生成物は、使用される特定の条件と試薬によって異なります。例えば、酸化によりリン酸誘導体が生成される一方、還元によりより単純な有機リン化合物が生成される可能性があります。
類似化合物との比較
Tributyl Phosphate: The precursor to Dibutyl-3-Hydroxybutyl Phosphate, used in similar industrial applications.
Dibutyl Phosphate: Another metabolite of tributyl phosphate with comparable properties.
Phosphoric Acid Esters: A broader class of compounds with similar chemical structures and reactivity.
Uniqueness: Dibutyl-3-Hydroxybutyl Phosphate is unique due to its specific metabolic origin and its distinct chemical properties. Its ability to undergo various chemical reactions and its wide range of applications in different fields make it a valuable compound for research and industrial use .
生物活性
Dibutyl 3-hydroxybutyl phosphate (DBHP) is an organophosphate compound with diverse biological activities. This article explores its biological effects, mechanisms of action, and relevant research findings, including case studies and data tables.
Dibutyl 3-hydroxybutyl phosphate is characterized by its structure, which includes a phosphate group linked to two butyl chains and a hydroxyl group. Its chemical formula is , and it is classified under organophosphates. The compound has been studied for its potential applications in various fields, including agriculture and medicine.
Antimicrobial Properties
DBHP has demonstrated antimicrobial activity against a range of pathogens. A study highlighted that DBHP derived from Streptomyces sp. exhibited significant inhibitory effects against human GSK-3β (glycogen synthase kinase 3 beta) and Plasmodium falciparum, the malaria parasite. The compound's mode of action involves molecular docking studies showing a higher binding affinity to the substrate-binding site of GSK-3β, suggesting a potential therapeutic role in malaria treatment .
Enzyme Inhibition
The enzyme inhibition studies revealed that DBHP acts as a mixed inhibitor of GSK-3β with an IC50 value of 2.0 µM. This indicates that DBHP can effectively inhibit the enzyme's activity, which is crucial in various cellular processes, including glycogen metabolism and cell signaling pathways. The mixed inhibition was characterized by changes in both and values during enzymatic assays, confirming its potential as a therapeutic agent .
Toxicity Studies
Toxicological evaluations have shown that DBHP has low acute toxicity levels in aquatic organisms, with no observed effects on fish, Daphnia, and algae at concentrations above 0.37 mg/L . Long-term exposure studies indicated that while high doses can lead to liver and urinary bladder changes in rodents, the compound does not exhibit genotoxic properties. This suggests that the observed effects are likely due to cytotoxicity rather than direct genetic damage .
Study on Hepatotoxicity
In a two-generation reproductive toxicity study involving Sprague-Dawley rats, DBHP was administered at varying concentrations (200 to 3,000 ppm). Results indicated no significant reproductive organ histopathology or effects on pre- or post-natal mortality at any dose level. However, higher concentrations resulted in increased liver weights and other mild changes in blood chemistry parameters .
Neurotoxicity Assessment
Neurotoxic potential was assessed through various studies; however, results were inconsistent. While some studies indicated cholinesterase inhibition at high doses, others found no neurological or behavioral changes associated with DBHP exposure . This discrepancy highlights the need for further investigation into the neurotoxic effects of DBHP.
Summary of Biological Activity Findings
Activity | Details |
---|---|
Antimicrobial | Inhibitory activity against Plasmodium falciparum and human GSK-3β |
Enzyme Inhibition | Mixed inhibition of GSK-3β with IC50 = 2.0 µM |
Aquatic Toxicity | NOEC for aquatic organisms at 0.37 mg/L |
Hepatotoxicity | Increased liver weight observed; no significant histopathological changes |
Neurotoxicity | Inconsistent results; some studies report cholinesterase inhibition |
特性
IUPAC Name |
dibutyl 3-hydroxybutyl phosphate | |
---|---|---|
Source | PubChem | |
URL | https://pubchem.ncbi.nlm.nih.gov | |
Description | Data deposited in or computed by PubChem | |
InChI |
InChI=1S/C12H27O5P/c1-4-6-9-15-18(14,16-10-7-5-2)17-11-8-12(3)13/h12-13H,4-11H2,1-3H3 | |
Source | PubChem | |
URL | https://pubchem.ncbi.nlm.nih.gov | |
Description | Data deposited in or computed by PubChem | |
InChI Key |
LJBNHONKIDIOPD-UHFFFAOYSA-N | |
Source | PubChem | |
URL | https://pubchem.ncbi.nlm.nih.gov | |
Description | Data deposited in or computed by PubChem | |
Canonical SMILES |
CCCCOP(=O)(OCCCC)OCCC(C)O | |
Source | PubChem | |
URL | https://pubchem.ncbi.nlm.nih.gov | |
Description | Data deposited in or computed by PubChem | |
Molecular Formula |
C12H27O5P | |
Source | PubChem | |
URL | https://pubchem.ncbi.nlm.nih.gov | |
Description | Data deposited in or computed by PubChem | |
DSSTOX Substance ID |
DTXSID901008696 | |
Record name | Dibutyl 3-hydroxybutyl phosphate | |
Source | EPA DSSTox | |
URL | https://comptox.epa.gov/dashboard/DTXSID901008696 | |
Description | DSSTox provides a high quality public chemistry resource for supporting improved predictive toxicology. | |
Molecular Weight |
282.31 g/mol | |
Source | PubChem | |
URL | https://pubchem.ncbi.nlm.nih.gov | |
Description | Data deposited in or computed by PubChem | |
CAS No. |
89197-69-3 | |
Record name | 4-Dibutoxyphosphoryloxybutan-2-ol | |
Source | ChemIDplus | |
URL | https://pubchem.ncbi.nlm.nih.gov/substance/?source=chemidplus&sourceid=0089197693 | |
Description | ChemIDplus is a free, web search system that provides access to the structure and nomenclature authority files used for the identification of chemical substances cited in National Library of Medicine (NLM) databases, including the TOXNET system. | |
Record name | Dibutyl 3-hydroxybutyl phosphate | |
Source | EPA DSSTox | |
URL | https://comptox.epa.gov/dashboard/DTXSID901008696 | |
Description | DSSTox provides a high quality public chemistry resource for supporting improved predictive toxicology. | |
Retrosynthesis Analysis
AI-Powered Synthesis Planning: Our tool employs the Template_relevance Pistachio, Template_relevance Bkms_metabolic, Template_relevance Pistachio_ringbreaker, Template_relevance Reaxys, Template_relevance Reaxys_biocatalysis model, leveraging a vast database of chemical reactions to predict feasible synthetic routes.
One-Step Synthesis Focus: Specifically designed for one-step synthesis, it provides concise and direct routes for your target compounds, streamlining the synthesis process.
Accurate Predictions: Utilizing the extensive PISTACHIO, BKMS_METABOLIC, PISTACHIO_RINGBREAKER, REAXYS, REAXYS_BIOCATALYSIS database, our tool offers high-accuracy predictions, reflecting the latest in chemical research and data.
Strategy Settings
Precursor scoring | Relevance Heuristic |
---|---|
Min. plausibility | 0.01 |
Model | Template_relevance |
Template Set | Pistachio/Bkms_metabolic/Pistachio_ringbreaker/Reaxys/Reaxys_biocatalysis |
Top-N result to add to graph | 6 |
Feasible Synthetic Routes
Q1: How is Dibutyl 3-hydroxybutyl phosphate formed in fish?
A1: Dibutyl 3-hydroxybutyl phosphate (DBHP) is a metabolite of Tributylphosphate (TBP), a commonly used alkyl organophosphate ester. Research has shown that fish, such as Carassius carassius (goldfish) and Oryzias latipes (killifish), can metabolize TBP into DBHP. This biotransformation process primarily occurs in the liver microsomes and is catalyzed by specific enzymes, mainly cytochrome P450 (CYP) enzymes, specifically CYP3A4 and CYP1A. [, ]
Q2: What is the significance of studying Dibutyl 3-hydroxybutyl phosphate formation?
A2: While DBHP itself might not be acutely toxic, understanding its formation and the metabolic pathways involved is crucial for several reasons.
- Biomarker of Exposure: The presence of DBHP in fish tissues can serve as a biomarker for Tributylphosphate exposure. [] This is important for monitoring environmental contamination and assessing potential risks to aquatic organisms.
- Species Differences: Researching metabolic pathways in different species helps us understand how various organisms process environmental contaminants and highlights potential differences in susceptibility. [] This knowledge is vital for developing effective conservation and risk management strategies.
試験管内研究製品の免責事項と情報
BenchChemで提示されるすべての記事および製品情報は、情報提供を目的としています。BenchChemで購入可能な製品は、生体外研究のために特別に設計されています。生体外研究は、ラテン語の "in glass" に由来し、生物体の外で行われる実験を指します。これらの製品は医薬品または薬として分類されておらず、FDAから任何の医療状態、病気、または疾患の予防、治療、または治癒のために承認されていません。これらの製品を人間または動物に体内に導入する形態は、法律により厳格に禁止されています。これらのガイドラインに従うことは、研究と実験において法的および倫理的な基準の遵守を確実にするために重要です。