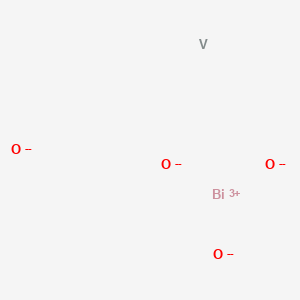
Bismuth vanadium tetraoxide
説明
Bismuth vanadium tetraoxide, also known as bismuth orthovanadate, is an inorganic compound with the chemical formula BiVO₄. It is a bright yellow solid that is widely studied for its photocatalytic properties, particularly in the field of environmental remediation. This compound is known for its narrow bandgap, resistance to corrosion, and low toxicity, making it an effective visible light-driven photocatalyst .
準備方法
Bismuth vanadium tetraoxide can be synthesized using various methods, including:
Sol-Gel Method: This method involves the hydrolysis and polycondensation of metal alkoxides.
Hydrothermal Method: This method uses high-temperature and high-pressure conditions to synthesize bismuth vanadium oxide from precursors like sodium bismuthate (NaBiO₃) and vanadium pentoxide (V₂O₅).
Precipitation Method: This involves pH-controlled precipitation reactions, which can be carried out with or without the presence of molybdenum, depending on the desired final phase.
化学反応の分析
Bismuth vanadium tetraoxide undergoes various chemical reactions, including:
Oxidation: It acts as a photocatalyst under visible light, facilitating the oxidation of organic pollutants in water.
Substitution: Doping with elements like tungsten and molybdenum can enhance its photocatalytic efficiency by altering its electronic structure.
Common reagents used in these reactions include hydrogen peroxide (H₂O₂) for oxidation and sodium borohydride (NaBH₄) for reduction. Major products formed from these reactions include degraded organic pollutants and reduced forms of bismuth vanadium oxide .
科学的研究の応用
Bismuth vanadium tetraoxide has a wide range of scientific research applications:
Environmental Remediation: It is used as a photocatalyst for the degradation of organic pollutants in water, including dyes and volatile organic compounds
Biomedical Applications: Research has shown its potential in drug delivery, antimicrobial activity, and cancer therapy.
Energy Storage: It is being explored for use in photoelectrochemical cells for hydrogen production.
Agriculture: Studies have demonstrated its ability to promote plant growth and inhibit plant pathogens.
作用機序
The photocatalytic activity of bismuth vanadium oxide is primarily due to its ability to generate electron-hole pairs under visible light irradiation. These electron-hole pairs can then participate in redox reactions, leading to the degradation of organic pollutants. The mechanism involves the absorption of photons, which excites electrons from the valence band to the conduction band, leaving behind holes in the valence band. These electrons and holes can then react with water and oxygen to produce reactive oxygen species (ROS) like hydroxyl radicals (•OH) and superoxide anions (O₂•−), which are responsible for the oxidation of pollutants .
類似化合物との比較
Bismuth vanadium tetraoxide can be compared with other similar compounds, such as:
Bismuth Tungstate (Bi₂WO₆): Both compounds are used as photocatalysts, but bismuth vanadium oxide has a narrower bandgap, making it more effective under visible light.
Bismuth Molybdate (Bi₂MoO₆): Similar to bismuth vanadium oxide, it is used in photocatalysis, but it has different electronic properties and photocatalytic efficiencies.
Bismuth Niobate (BiNbO₄): This compound is also used in photocatalysis and has unique structural properties compared to bismuth vanadium oxide.
This compound stands out due to its unique combination of narrow bandgap, low toxicity, and high resistance to corrosion, making it a versatile and effective photocatalyst for various applications.
特性
IUPAC Name |
bismuth;oxygen(2-);vanadium | |
---|---|---|
Source | PubChem | |
URL | https://pubchem.ncbi.nlm.nih.gov | |
Description | Data deposited in or computed by PubChem | |
InChI |
InChI=1S/Bi.4O.V/q+3;4*-2; | |
Source | PubChem | |
URL | https://pubchem.ncbi.nlm.nih.gov | |
Description | Data deposited in or computed by PubChem | |
InChI Key |
HUUOUJVWIOKBMD-UHFFFAOYSA-N | |
Source | PubChem | |
URL | https://pubchem.ncbi.nlm.nih.gov | |
Description | Data deposited in or computed by PubChem | |
Canonical SMILES |
[O-2].[O-2].[O-2].[O-2].[V].[Bi+3] | |
Source | PubChem | |
URL | https://pubchem.ncbi.nlm.nih.gov | |
Description | Data deposited in or computed by PubChem | |
Molecular Formula |
BiO4V-5 | |
Source | PubChem | |
URL | https://pubchem.ncbi.nlm.nih.gov | |
Description | Data deposited in or computed by PubChem | |
Molecular Weight |
323.919 g/mol | |
Source | PubChem | |
URL | https://pubchem.ncbi.nlm.nih.gov | |
Description | Data deposited in or computed by PubChem | |
CAS No. |
14059-33-7, 53801-77-7 | |
Record name | Bismuth vanadium tetraoxide | |
Source | ChemIDplus | |
URL | https://pubchem.ncbi.nlm.nih.gov/substance/?source=chemidplus&sourceid=0014059337 | |
Description | ChemIDplus is a free, web search system that provides access to the structure and nomenclature authority files used for the identification of chemical substances cited in National Library of Medicine (NLM) databases, including the TOXNET system. | |
Record name | Bismuth orthovanadate | |
Source | ChemIDplus | |
URL | https://pubchem.ncbi.nlm.nih.gov/substance/?source=chemidplus&sourceid=0053801777 | |
Description | ChemIDplus is a free, web search system that provides access to the structure and nomenclature authority files used for the identification of chemical substances cited in National Library of Medicine (NLM) databases, including the TOXNET system. | |
Record name | Bismuth vanadium tetraoxide | |
Source | European Chemicals Agency (ECHA) | |
URL | https://echa.europa.eu/substance-information/-/substanceinfo/100.034.439 | |
Description | The European Chemicals Agency (ECHA) is an agency of the European Union which is the driving force among regulatory authorities in implementing the EU's groundbreaking chemicals legislation for the benefit of human health and the environment as well as for innovation and competitiveness. | |
Explanation | Use of the information, documents and data from the ECHA website is subject to the terms and conditions of this Legal Notice, and subject to other binding limitations provided for under applicable law, the information, documents and data made available on the ECHA website may be reproduced, distributed and/or used, totally or in part, for non-commercial purposes provided that ECHA is acknowledged as the source: "Source: European Chemicals Agency, http://echa.europa.eu/". Such acknowledgement must be included in each copy of the material. ECHA permits and encourages organisations and individuals to create links to the ECHA website under the following cumulative conditions: Links can only be made to webpages that provide a link to the Legal Notice page. | |
Record name | Bismuth vanadium oxide | |
Source | European Chemicals Agency (ECHA) | |
URL | https://echa.europa.eu/substance-information/-/substanceinfo/100.053.424 | |
Description | The European Chemicals Agency (ECHA) is an agency of the European Union which is the driving force among regulatory authorities in implementing the EU's groundbreaking chemicals legislation for the benefit of human health and the environment as well as for innovation and competitiveness. | |
Explanation | Use of the information, documents and data from the ECHA website is subject to the terms and conditions of this Legal Notice, and subject to other binding limitations provided for under applicable law, the information, documents and data made available on the ECHA website may be reproduced, distributed and/or used, totally or in part, for non-commercial purposes provided that ECHA is acknowledged as the source: "Source: European Chemicals Agency, http://echa.europa.eu/". Such acknowledgement must be included in each copy of the material. ECHA permits and encourages organisations and individuals to create links to the ECHA website under the following cumulative conditions: Links can only be made to webpages that provide a link to the Legal Notice page. | |
試験管内研究製品の免責事項と情報
BenchChemで提示されるすべての記事および製品情報は、情報提供を目的としています。BenchChemで購入可能な製品は、生体外研究のために特別に設計されています。生体外研究は、ラテン語の "in glass" に由来し、生物体の外で行われる実験を指します。これらの製品は医薬品または薬として分類されておらず、FDAから任何の医療状態、病気、または疾患の予防、治療、または治癒のために承認されていません。これらの製品を人間または動物に体内に導入する形態は、法律により厳格に禁止されています。これらのガイドラインに従うことは、研究と実験において法的および倫理的な基準の遵守を確実にするために重要です。