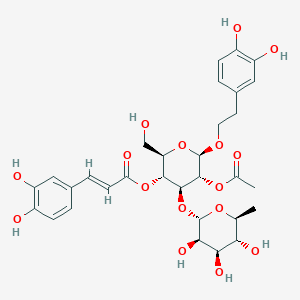
2-Acetylacteoside
概要
説明
2-Acetylacteoside, also referred to as 2'-acetylacteoside, is a phenylethanoid glycoside (PhG) that has been isolated from various plants, including Cistanches salsa and Cistanche deserticola. It has garnered attention due to its pharmacological properties and potential health benefits. The compound has been shown to possess significant anti-osteoporotic effects, as demonstrated in studies involving ovariectomized mice, where it improved bone mineral density and micro-architecture . Additionally, 2-acetylacteoside has been associated with anti-inflammatory activities, particularly in the context of vascular endothelial cells, where it downregulated cell adhesion molecules involved in atherosclerosis and inflammation .
Synthesis Analysis
The isolation and purification of 2-acetylacteoside have been successfully achieved using high-speed counter-current chromatography (HSCCC) from Cistanches salsa. A quaternary two-phase solvent system was utilized, resulting in the extraction of 2-acetylacteoside with over 98% purity, as confirmed by HPLC analyses. The chemical structures of the purified compounds were identified using 1H NMR, 13C NMR, and MS techniques .
Molecular Structure Analysis
The molecular structure of 2-acetylacteoside was elucidated through nuclear magnetic resonance (NMR) and mass spectrometry (MS). These advanced analytical techniques allowed for the detailed identification of the compound's structure, which is crucial for understanding its biological activity and interactions with other molecules .
Chemical Reactions Analysis
While the specific chemical reactions involving 2-acetylacteoside have not been detailed in the provided papers, the compound's ability to modulate biological pathways suggests that it may interact with various enzymes and receptors. For instance, its anti-osteoporotic activity is thought to be mediated through the RANKL/RANK/TRAF6-mediated NF-κB/NFATc1 pathway, indicating a complex interplay of biochemical reactions .
Physical and Chemical Properties Analysis
The physical and chemical properties of 2-acetylacteoside, such as solubility, stability, and reactivity, are inferred from its successful isolation using HSCCC and its biological activities. The high purity of the compound suggests that it has distinct physical properties that allow for its separation from other components. Its chemical properties, particularly its interactions with cellular pathways, are indicative of its potential as a therapeutic agent .
科学的研究の応用
Metabolic Profiling and Bioactivity
- Metabolism by Human Intestinal Bacteria : 2'-Acetylacteoside undergoes multiple metabolic processes in the gastrointestinal tract. It transforms into bioactive metabolites like hydroxytyrosol and 3-hydroxyphenylpropionic, which exhibit similar bioactivities to their precursors. The study of these metabolites is crucial for understanding the pharmacokinetics of phenylethanoid glycosides (PhGs) (Li et al., 2016).
Therapeutic Potential
- Anti-Osteoporotic Effects : 2'-Acetylacteoside demonstrates significant anti-osteoporotic effects in ovariectomized mice, suggesting its potential as an anti-osteoporotic candidate. It affects bone resorption markers and operates through the RANKL/RANK/TRAF6-mediated NF-κB/NFATc1 pathway (Li et al., 2020).
- Inhibition of Cell Adhesion Molecules : 2'-Acetylacteoside inhibits the expression of cell adhesion molecules (CAMs) in vascular endothelial cells, indicating potential anti-inflammatory activities in vascular endothelium (Chen et al., 2009).
- Anti-proliferative Effects on Aortic Smooth Muscle : Exhibits concentration-dependent suppression of the proliferative response of rat aortic smooth muscle cells, indicating potential preventative effects on arteriosclerosis (He et al., 2001).
Antioxidant and Photoprotective Properties
- Antioxidant and Antihypertensive Activities : Demonstrates effective scavenging of radicals and inhibits ACE activity. Acteoside, a related compound, shows antihypertensive activity in vivo, suggesting potential for blood pressure regulation (Chen et al., 2012).
- Photoprotective Effects Against UV Radiation-Induced Skin Damage : Acteoside and 2'-acetylacteoside reduce UVB-induced MMP-1 expression and stimulate type I procollagen synthesis, indicating potential in treating skin photodamage (Gao et al., 2021).
Chemical Analysis and Cultivation
- Chemical Diversity in Cistanche Herbs : 2′-Acetylacteoside is present in Cistanche deserticola and can be a potential chemical marker for this species. It's also useful in assessing the quality of herbal medicine (Wang et al., 2019).
- Isolation and Purification Techniques : High-speed counter-current chromatography (HSCCC) has been used for the isolation and purification of 2'-acetylacteoside from plants like Cistanche deserticola, aiding in the study of its properties and applications (Li et al., 2008).
Neuroprotective Activities
- Neuroprotection Against Glutamate-Induced Neurotoxicity : Shows significant neuroprotective activities in primary cultures of rat cortical cells, indicating potential benefits in neurological disorders or injuries (Koo et al., 2005).
作用機序
Target of Action
2-Acetylacteoside is a phenylethanoid glycoside that has been found in C. deserticola . It selectively inhibits aldose reductase , an enzyme involved in the polyol pathway of glucose metabolism. This enzyme plays a crucial role in the development of long-term complications of diabetes mellitus .
Mode of Action
2-Acetylacteoside interacts with its target, aldose reductase, by selectively inhibiting its activity . This inhibition prevents the conversion of glucose to sorbitol, a process that can lead to harmful effects when uncontrolled, especially in the context of hyperglycemia in diabetes .
Biochemical Pathways
The primary biochemical pathway affected by 2-Acetylacteoside is the polyol pathway of glucose metabolism . By inhibiting aldose reductase, 2-Acetylacteoside prevents the accumulation of sorbitol, which can cause osmotic stress and contribute to diabetic complications .
Result of Action
The inhibition of aldose reductase by 2-Acetylacteoside can prevent the accumulation of sorbitol, thereby mitigating osmotic stress and potential cellular damage . This can be particularly beneficial in the context of diabetes, where uncontrolled glucose levels can lead to an overactive polyol pathway and subsequent cellular damage .
Safety and Hazards
特性
IUPAC Name |
[(2R,3R,4S,5R,6R)-5-acetyloxy-6-[2-(3,4-dihydroxyphenyl)ethoxy]-2-(hydroxymethyl)-4-[(2S,3R,4R,5R,6S)-3,4,5-trihydroxy-6-methyloxan-2-yl]oxyoxan-3-yl] (E)-3-(3,4-dihydroxyphenyl)prop-2-enoate | |
---|---|---|
Source | PubChem | |
URL | https://pubchem.ncbi.nlm.nih.gov | |
Description | Data deposited in or computed by PubChem | |
InChI |
InChI=1S/C31H38O16/c1-14-24(39)25(40)26(41)30(43-14)47-28-27(46-23(38)8-5-16-3-6-18(34)20(36)11-16)22(13-32)45-31(29(28)44-15(2)33)42-10-9-17-4-7-19(35)21(37)12-17/h3-8,11-12,14,22,24-32,34-37,39-41H,9-10,13H2,1-2H3/b8-5+/t14-,22+,24-,25+,26+,27+,28-,29+,30-,31+/m0/s1 | |
Source | PubChem | |
URL | https://pubchem.ncbi.nlm.nih.gov | |
Description | Data deposited in or computed by PubChem | |
InChI Key |
ALERZNQPBWWLMW-OMRKUVHCSA-N | |
Source | PubChem | |
URL | https://pubchem.ncbi.nlm.nih.gov | |
Description | Data deposited in or computed by PubChem | |
Canonical SMILES |
CC1C(C(C(C(O1)OC2C(C(OC(C2OC(=O)C)OCCC3=CC(=C(C=C3)O)O)CO)OC(=O)C=CC4=CC(=C(C=C4)O)O)O)O)O | |
Source | PubChem | |
URL | https://pubchem.ncbi.nlm.nih.gov | |
Description | Data deposited in or computed by PubChem | |
Isomeric SMILES |
C[C@H]1[C@@H]([C@H]([C@H]([C@@H](O1)O[C@H]2[C@@H]([C@H](O[C@H]([C@@H]2OC(=O)C)OCCC3=CC(=C(C=C3)O)O)CO)OC(=O)/C=C/C4=CC(=C(C=C4)O)O)O)O)O | |
Source | PubChem | |
URL | https://pubchem.ncbi.nlm.nih.gov | |
Description | Data deposited in or computed by PubChem | |
Molecular Formula |
C31H38O16 | |
Source | PubChem | |
URL | https://pubchem.ncbi.nlm.nih.gov | |
Description | Data deposited in or computed by PubChem | |
Molecular Weight |
666.6 g/mol | |
Source | PubChem | |
URL | https://pubchem.ncbi.nlm.nih.gov | |
Description | Data deposited in or computed by PubChem | |
Product Name |
2-Acetylacteoside |
Retrosynthesis Analysis
AI-Powered Synthesis Planning: Our tool employs the Template_relevance Pistachio, Template_relevance Bkms_metabolic, Template_relevance Pistachio_ringbreaker, Template_relevance Reaxys, Template_relevance Reaxys_biocatalysis model, leveraging a vast database of chemical reactions to predict feasible synthetic routes.
One-Step Synthesis Focus: Specifically designed for one-step synthesis, it provides concise and direct routes for your target compounds, streamlining the synthesis process.
Accurate Predictions: Utilizing the extensive PISTACHIO, BKMS_METABOLIC, PISTACHIO_RINGBREAKER, REAXYS, REAXYS_BIOCATALYSIS database, our tool offers high-accuracy predictions, reflecting the latest in chemical research and data.
Strategy Settings
Precursor scoring | Relevance Heuristic |
---|---|
Min. plausibility | 0.01 |
Model | Template_relevance |
Template Set | Pistachio/Bkms_metabolic/Pistachio_ringbreaker/Reaxys/Reaxys_biocatalysis |
Top-N result to add to graph | 6 |
Feasible Synthetic Routes
Q & A
Q1: How does 2'-acetylacteoside exert its anti-cancer effects?
A1: 2'-Acetylacteoside exhibits anti-cancer activity through multiple mechanisms. In T-cell lymphoma cells, it induces both apoptosis and pyroptosis. [] This compound elevates p53 levels by inhibiting the SIRT2-MDM2/P300 and PI3K/AKT signaling pathways while activating the PTEN-Bax pathway. [] Additionally, 2'-acetylacteoside triggers pyroptosis through non-canonical and alternative pathways, suppressing the NLRP3 inflammasome and IL-1β maturation. []
Q2: Does 2'-acetylacteoside impact melanogenesis?
A2: Yes, 2'-acetylacteoside demonstrates significant inhibitory effects on melanin synthesis in human epidermal melanocytes. [] It achieves this by reducing tyrosinase activity in a dose-dependent manner. [] The presence of phenolic hydroxyl groups and aglycone, steric hindrance, substituents on the middle glucopyranose, and the location of phenolic hydroxyl all influence its inhibitory potency. []
Q3: What are the neuroprotective effects of 2'-acetylacteoside?
A3: 2'-Acetylacteoside displays neuroprotective properties by significantly attenuating glutamate-induced neurotoxicity in rat cortical cell cultures. [] This effect is observed at concentrations ranging from 0.1 to 10 μM. []
Q4: What is the molecular formula and weight of 2'-acetylacteoside?
A4: While a specific molecular formula and weight are not explicitly mentioned in the provided abstracts, 2'-acetylacteoside's structure is described as a phenylethanoid glycoside. These compounds are characterized by a phenylethyl moiety linked to a glycoside unit. Further spectroscopic data would be required for complete structural elucidation.
Q5: What spectroscopic techniques are commonly used to characterize 2'-acetylacteoside?
A5: Researchers often employ a combination of spectroscopic methods for structural analysis, including:
- High-resolution mass spectrometry (HR-MS): Used to determine the molecular mass and fragmentation patterns, providing insights into the compound's structure and potential modifications. [, , , ]
- Nuclear Magnetic Resonance (NMR) spectroscopy: Both 1H-NMR and 13C-NMR are crucial for elucidating the compound's structure, providing information about the type, number, and arrangement of atoms within the molecule. []
Q6: How does steaming with wine affect the content of 2'-acetylacteoside in Cistanche deserticola?
A6: Steaming Cistanche deserticola with wine leads to a decrease in 2'-acetylacteoside content as the steaming time increases. [] This suggests potential degradation or transformation of the compound under these specific processing conditions.
Q7: Does the season of harvest impact the 2'-acetylacteoside content in Cistanche deserticola?
A7: Yes, the content of 2'-acetylacteoside in Cistanche deserticola varies depending on the season of harvest. [] This highlights the potential influence of environmental factors and plant growth stages on the production of secondary metabolites like 2'-acetylacteoside.
Q8: How do structural modifications affect the antioxidant activity of 2'-acetylacteoside and related compounds?
A8: The antioxidant activity of phenylethanoid glycosides, including 2'-acetylacteoside, is influenced by several structural factors:
- Number of phenolic hydroxyl groups: A higher number of phenolic hydroxyl groups generally enhances antioxidant activity. [, ]
- Steric hindrance: Bulky substituents near the phenolic hydroxyl groups can hinder their interaction with free radicals, potentially reducing antioxidant activity. []
- Presence of 2-acetyl group: The presence of a 2-acetyl group on the middle glucopyranose may enhance antioxidant activity, as observed with 2'-acetylacteoside compared to acteoside. []
- Location of phenolic hydroxyl groups: The position of phenolic hydroxyl groups within the molecule can influence their electron-donating ability and, consequently, their antioxidant potency. []
Q9: What evidence supports the hepatoprotective activity of 2'-acetylacteoside?
A9: Studies have demonstrated the hepatoprotective effects of 2'-acetylacteoside and other phenylethanoids from Cistanche deserticola. These compounds suppress NADPH/CCl4-induced lipid peroxidation in rat liver microsomes and protect primary cultured rat hepatocytes from CCl4 or D-galactosamine-induced damage. []
Q10: What analytical techniques are used to quantify 2'-acetylacteoside in plant materials?
A10: High-performance liquid chromatography (HPLC) coupled with various detection methods is widely used for the quantification of 2'-acetylacteoside:
- HPLC-ELSD: High-performance liquid chromatography coupled with evaporative light scattering detection (ELSD) is utilized for the isolation and purification of 2'-acetylacteoside from Cistanche deserticola. []
- HPLC-DAD: High-performance liquid chromatography coupled with diode array detection (DAD) enables the simultaneous determination of multiple phenylethanoid glycosides, including 2'-acetylacteoside, in Cistanche species. []
Q11: How do researchers ensure the quality control of Cistanche herbs containing 2'-acetylacteoside?
A11: Quality control of Cistanche herbs involves various measures:
- Chemical profiling: Techniques like UPLC-PDA-Q/TOF-MS help establish the chemical composition of different Cistanche species and identify potential adulterants. []
- Quantification of marker compounds: Accurate determination of 2'-acetylacteoside and other key phenylethanoid glycosides using validated analytical methods ensures batch-to-batch consistency. [, ]
試験管内研究製品の免責事項と情報
BenchChemで提示されるすべての記事および製品情報は、情報提供を目的としています。BenchChemで購入可能な製品は、生体外研究のために特別に設計されています。生体外研究は、ラテン語の "in glass" に由来し、生物体の外で行われる実験を指します。これらの製品は医薬品または薬として分類されておらず、FDAから任何の医療状態、病気、または疾患の予防、治療、または治癒のために承認されていません。これらの製品を人間または動物に体内に導入する形態は、法律により厳格に禁止されています。これらのガイドラインに従うことは、研究と実験において法的および倫理的な基準の遵守を確実にするために重要です。