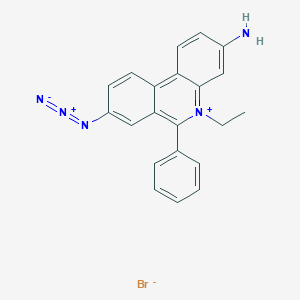
Ethidium monoazide bromide
概要
説明
Ethidium Monoazide Bromide (EMA) is a photo-activated fluorescent nucleic acid binding dye . It is used to selectively and covalently label dead cells in the presence of live cells for viability PCR, fluorescent microscopy, and flow cytometry . When not activated or bound to DNA, EMA is a non-fluorescent nucleic acid stain with a photoaffinity label . Once photoactivated, the dye binds covalently to nucleic acids and can emit fluorescence .
Physical And Chemical Properties Analysis
EMA has a molecular weight of 420.3 g/mol . It has a weak fluorescence which increases approximately 15-fold on binding to DNA with excitation/emission maxima of approximately 504/600 nm .
科学的研究の応用
DNA Amplification Suppression
EMA has been determined to cause delay in DNA amplification from dead bacteria at real-time PCR . This property is particularly useful in food hygiene tests where it’s important to distinguish between live and dead bacteria .
Detection of Live Bacteria
A novel application of EMA is in the detection of live bacteria in powdered infant formula . A low-dose double EMA treatment was developed to increase the distinction ability between live and dead bacteria .
Fluorescent Photoaffinity Label
EMA is a fluorescent photoaffinity label that binds covalently to nucleic acids both in solution and in cells that have compromised membranes . The fluorescence of EMA is weak, but the intensity increases approximately 15-fold on binding to DNA .
Footprinting Drug Binding Sites on DNA
EMA has been used to footprint drug binding sites on DNA . This application is crucial in understanding how drugs interact with DNA at a molecular level.
Detection of Non-Viable Cells
EMA is used to detect non-viable cells . This is particularly useful in research fields where the viability of cells is a critical factor.
Identification of Ethidium Binding Sites on DNA and tRNA
EMA is used to identify ethidium binding sites on DNA and tRNA . This helps in understanding the interaction between ethidium and nucleic acids.
Selective Inactivation of Gene Expression
EMA has been used to selectively inactivate gene expression . This application is important in genetic research and gene therapy.
Labeling Yeast Cells for Leukocyte Phagocytosis Assays
EMA has been used for labeling yeast cells for leukocyte phagocytosis assays . This application is significant in immunology research, particularly in studying the immune response.
作用機序
Target of Action
Ethidium Monoazide Bromide (EMA) primarily targets nucleic acids , both in solution and in cells that have compromised membranes . It is a fluorescent photoaffinity label that, after photolysis, binds covalently to nucleic acids .
Mode of Action
EMA is a photoactive stain that covalently binds to nucleic acids in solution and in cells with damaged membranes . After photolysis, EMA binds covalently to nucleic acids . The fluorescence of EMA is weak, but the intensity increases approximately 15-fold on binding to DNA .
Biochemical Pathways
It is known that ema can be used to footprint drug binding sites on dna, detect non-viable cells, identify ethidium binding sites on dna and trna, and selectively inactivate gene expression .
Pharmacokinetics
It is known that ema is relatively impermeant to live cells, selectively binding dna in dead cells .
Result of Action
The action of EMA results in the covalent binding of the compound to the nucleic acids of cells with compromised membranes . This binding enhances the fluorescence intensity of EMA, making it a useful tool for identifying non-viable cells .
Action Environment
The action of EMA is influenced by light, as photolysis is required for EMA to bind covalently to nucleic acids . Furthermore, EMA is relatively impermeant to live cells, suggesting that the integrity of the cell membrane can influence the action of EMA .
Safety and Hazards
While specific safety and hazards information for EMA is not available, it is known that Ethidium Bromide, a related compound, is highly toxic. It can be absorbed through the skin and is highly toxic by inhalation. It is also irritating to the skin, eyes, mucous membranes, and upper respiratory tract .
将来の方向性
特性
IUPAC Name |
8-azido-5-ethyl-6-phenylphenanthridin-5-ium-3-amine;bromide | |
---|---|---|
Source | PubChem | |
URL | https://pubchem.ncbi.nlm.nih.gov | |
Description | Data deposited in or computed by PubChem | |
InChI |
InChI=1S/C21H17N5.BrH/c1-2-26-20-12-15(22)8-10-18(20)17-11-9-16(24-25-23)13-19(17)21(26)14-6-4-3-5-7-14;/h3-13,22H,2H2,1H3;1H | |
Source | PubChem | |
URL | https://pubchem.ncbi.nlm.nih.gov | |
Description | Data deposited in or computed by PubChem | |
InChI Key |
GHUXAYLZEGLXDA-UHFFFAOYSA-N | |
Source | PubChem | |
URL | https://pubchem.ncbi.nlm.nih.gov | |
Description | Data deposited in or computed by PubChem | |
Canonical SMILES |
CC[N+]1=C2C=C(C=CC2=C3C=CC(=CC3=C1C4=CC=CC=C4)N=[N+]=[N-])N.[Br-] | |
Source | PubChem | |
URL | https://pubchem.ncbi.nlm.nih.gov | |
Description | Data deposited in or computed by PubChem | |
Molecular Formula |
C21H18BrN5 | |
Source | PubChem | |
URL | https://pubchem.ncbi.nlm.nih.gov | |
Description | Data deposited in or computed by PubChem | |
Related CAS |
69498-50-6 (Parent) | |
Record name | Phenanthridinium, 3-amino-8-azido-5-ethyl-6-phenyl-, bromide | |
Source | ChemIDplus | |
URL | https://pubchem.ncbi.nlm.nih.gov/substance/?source=chemidplus&sourceid=0058880050 | |
Description | ChemIDplus is a free, web search system that provides access to the structure and nomenclature authority files used for the identification of chemical substances cited in National Library of Medicine (NLM) databases, including the TOXNET system. | |
Molecular Weight |
420.3 g/mol | |
Source | PubChem | |
URL | https://pubchem.ncbi.nlm.nih.gov | |
Description | Data deposited in or computed by PubChem | |
Product Name |
Ethidium monoazide bromide | |
CAS RN |
58880-05-0 | |
Record name | Ethidium monoazide bromide | |
Source | CAS Common Chemistry | |
URL | https://commonchemistry.cas.org/detail?cas_rn=58880-05-0 | |
Description | CAS Common Chemistry is an open community resource for accessing chemical information. Nearly 500,000 chemical substances from CAS REGISTRY cover areas of community interest, including common and frequently regulated chemicals, and those relevant to high school and undergraduate chemistry classes. This chemical information, curated by our expert scientists, is provided in alignment with our mission as a division of the American Chemical Society. | |
Explanation | The data from CAS Common Chemistry is provided under a CC-BY-NC 4.0 license, unless otherwise stated. | |
Record name | Phenanthridinium, 3-amino-8-azido-5-ethyl-6-phenyl-, bromide | |
Source | ChemIDplus | |
URL | https://pubchem.ncbi.nlm.nih.gov/substance/?source=chemidplus&sourceid=0058880050 | |
Description | ChemIDplus is a free, web search system that provides access to the structure and nomenclature authority files used for the identification of chemical substances cited in National Library of Medicine (NLM) databases, including the TOXNET system. | |
Retrosynthesis Analysis
AI-Powered Synthesis Planning: Our tool employs the Template_relevance Pistachio, Template_relevance Bkms_metabolic, Template_relevance Pistachio_ringbreaker, Template_relevance Reaxys, Template_relevance Reaxys_biocatalysis model, leveraging a vast database of chemical reactions to predict feasible synthetic routes.
One-Step Synthesis Focus: Specifically designed for one-step synthesis, it provides concise and direct routes for your target compounds, streamlining the synthesis process.
Accurate Predictions: Utilizing the extensive PISTACHIO, BKMS_METABOLIC, PISTACHIO_RINGBREAKER, REAXYS, REAXYS_BIOCATALYSIS database, our tool offers high-accuracy predictions, reflecting the latest in chemical research and data.
Strategy Settings
Precursor scoring | Relevance Heuristic |
---|---|
Min. plausibility | 0.01 |
Model | Template_relevance |
Template Set | Pistachio/Bkms_metabolic/Pistachio_ringbreaker/Reaxys/Reaxys_biocatalysis |
Top-N result to add to graph | 6 |
Feasible Synthetic Routes
試験管内研究製品の免責事項と情報
BenchChemで提示されるすべての記事および製品情報は、情報提供を目的としています。BenchChemで購入可能な製品は、生体外研究のために特別に設計されています。生体外研究は、ラテン語の "in glass" に由来し、生物体の外で行われる実験を指します。これらの製品は医薬品または薬として分類されておらず、FDAから任何の医療状態、病気、または疾患の予防、治療、または治癒のために承認されていません。これらの製品を人間または動物に体内に導入する形態は、法律により厳格に禁止されています。これらのガイドラインに従うことは、研究と実験において法的および倫理的な基準の遵守を確実にするために重要です。