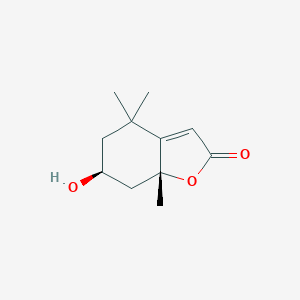
Loliolide
概要
説明
Loliolide is a naturally occurring monoterpenoid hydroxyl lactone found in various plant species and marine algae. It is known for its diverse biological activities, including antioxidant, anti-inflammatory, and neuroprotective properties . This compound has garnered significant interest in scientific research due to its potential therapeutic applications.
準備方法
Synthetic Routes and Reaction Conditions: Loliolide can be synthesized through various methods. One common approach involves the extraction from natural sources such as the green seaweed Codium tomentosum. The extraction process typically involves the use of diethyl ether, followed by purification through column chromatography and identification by nuclear magnetic resonance spectroscopy . Another method involves the use of amine-based microporous organic polymers for solid-phase extraction, followed by macroporous resin chromatography and preparative high-performance liquid chromatography .
Industrial Production Methods: Industrial production of this compound often involves large-scale extraction from marine algae or other plant sources. The process includes solid-phase extraction, macroporous resin chromatography, and high-performance liquid chromatography to obtain high-purity this compound .
化学反応の分析
Types of Reactions: Loliolide undergoes various chemical reactions, including oxidation, reduction, and substitution.
Common Reagents and Conditions:
Oxidation: this compound can be oxidized using reagents such as potassium permanganate or hydrogen peroxide under acidic or basic conditions.
Reduction: Reduction of this compound can be achieved using reagents like sodium borohydride or lithium aluminum hydride.
Substitution: Substitution reactions involving this compound often use halogenating agents or nucleophiles under controlled conditions.
Major Products Formed: The major products formed from these reactions include oxidized derivatives, reduced forms of this compound, and substituted this compound compounds with various functional groups .
科学的研究の応用
Neuroprotective Effects
Research indicates that loliolide exhibits significant neuroprotective properties. A study demonstrated that this compound could enhance cell viability against neurotoxic agents like 6-hydroxydopamine (6-OHDA) in SH-SY5Y cells, suggesting its potential as a therapeutic agent for neurological diseases such as Parkinson's disease. The mechanism involves mitochondrial protection, reduction of oxidative stress, and inhibition of pro-inflammatory cytokines like TNF-α and IL-6 .
Anti-inflammatory Properties
This compound has shown promise in reducing inflammation. In human retinal pigment epithelial (RPE) cells exposed to ultrafine particulate matter, this compound significantly alleviated inflammation by inhibiting the MAPK/NF-κB signaling pathways . Additionally, it suppressed nitric oxide production in macrophages, further supporting its anti-inflammatory potential .
Antioxidant Activity
The compound's antioxidant properties are well-documented. Isolated from Sargassum ringgoldianum, this compound demonstrated protective effects against hydrogen peroxide-induced cell damage through various assays, including DPPH and H2O2 radical scavenging tests . This suggests its application in preventing oxidative stress-related diseases.
Cosmetic Applications
This compound's anti-aging and wound-healing properties make it a valuable ingredient in the cosmetic industry. Studies on human keratinocyte cells (HaCaT) revealed that this compound could inhibit apoptosis induced by UV radiation and promote skin repair by enhancing the expression of growth factors and reducing matrix metalloproteinase levels associated with wrinkle formation . These findings highlight its potential use in skincare formulations aimed at anti-aging and skin regeneration.
Anti-adipogenic Effects
Recent studies have shown that this compound can inhibit adipogenesis in pre-adipocytes (3T3-L1 cells). It significantly reduced lipid accumulation and promoted lipolysis by enhancing the expression of thermogenic markers such as PGC-1α and hormone-sensitive lipase (HSL) . This suggests a potential role for this compound in weight management and metabolic health.
Diabetes Management
The compound's ability to regulate lipid metabolism may extend to diabetes management, where controlling adipose tissue development is crucial for insulin sensitivity and overall metabolic health .
Case Studies and Research Findings
作用機序
類似化合物との比較
- Dihydroactinidiolide
- β-Cyclocitral
- Epiloliolide
This compound stands out due to its diverse range of applications and potent biological activities, making it a compound of significant interest in various fields of research.
生物活性
Loliolide is a monoterpenoid hydroxylactone predominantly found in various algae and plants. Its biological activities have garnered significant interest due to its potential therapeutic applications, particularly in neuroprotection, anti-inflammation, and anti-apoptosis. This article provides an overview of the biological activity of this compound, supported by research findings, data tables, and case studies.
Chemical Structure and Properties
This compound has a simple structure characterized by a hydroxyl group and a lactone ring. Its chemical formula is , which contributes to its diverse biological activities. The compound is often isolated from sources such as Cladophora tomentosum and Sargassum ringgoldianum.
Neuroprotective Effects
Recent studies have highlighted this compound's neuroprotective properties, particularly against neurotoxic agents like 6-hydroxydopamine (6-OHDA). In vitro experiments using SH-SY5Y neuronal cells demonstrated that this compound significantly increased cell viability and reduced oxidative stress markers:
Concentration (µM) | Cell Viability Increase (%) | ROS Levels Decrease (%) | Caspase-3 Activity Decrease (%) |
---|---|---|---|
50 | 23.70 ± 7.77 | 35.4 | 110.1 ± 37.82 |
100 | 41.06 ± 6.31 | 45.0 | 126.1 ± 48.95 |
This compound's mechanism of action includes mitochondrial protection, reduction of reactive oxygen species (ROS), and inhibition of the NF-kB pathway, which is crucial in inflammation and apoptosis regulation .
Anti-inflammatory Activity
This compound exhibits significant anti-inflammatory effects by suppressing the production of pro-inflammatory cytokines such as TNF-α and IL-6 in RAW 264.7 macrophages. The compound also inhibits nitric oxide production, further demonstrating its potential as an anti-inflammatory agent .
Antioxidant Properties
The antioxidant capacity of this compound has been evaluated through various assays, indicating its ability to scavenge free radicals and protect cellular components from oxidative damage. Studies have shown that treatment with this compound enhances catalase activity, which is vital for detoxifying hydrogen peroxide in cells:
Treatment Condition | Catalase Activity Increase (%) |
---|---|
Control | 24.00 ± 1.03 |
This compound (100 µM) | 60.40 ± 3.72 |
This increase in catalase activity suggests that this compound not only protects against oxidative stress but also enhances the cellular antioxidant defense system .
Anti-apoptotic Effects
In addition to its neuroprotective and anti-inflammatory properties, this compound has shown promising results in preventing apoptosis in human keratinocytes (HaCaT cells) exposed to UV radiation. The compound reduced ROS levels and DNA damage while decreasing the expression of apoptotic markers such as caspases:
Treatment Condition | Cell Death Reduction (%) |
---|---|
UVB Only | 70% |
UVB + this compound (100 µM) | 30% |
These findings indicate that this compound can mitigate UV-induced cell death through its antioxidant effects .
Case Studies
- Neuroprotective Study : A study investigated the effects of this compound on SH-SY5Y cells treated with 6-OHDA, revealing that this compound significantly improved cell viability and reduced apoptotic markers compared to untreated controls .
- Anti-inflammatory Research : Another study focused on this compound's effects on RAW 264.7 macrophages, demonstrating a marked reduction in nitric oxide production and inflammatory cytokines upon treatment with the compound .
- Photoprotective Effects : Research on HaCaT cells showed that pretreatment with this compound before UV exposure led to decreased oxidative stress and enhanced cell survival rates, suggesting its potential use in photoprotection strategies .
特性
IUPAC Name |
(6S,7aR)-6-hydroxy-4,4,7a-trimethyl-6,7-dihydro-5H-1-benzofuran-2-one | |
---|---|---|
Source | PubChem | |
URL | https://pubchem.ncbi.nlm.nih.gov | |
Description | Data deposited in or computed by PubChem | |
InChI |
InChI=1S/C11H16O3/c1-10(2)5-7(12)6-11(3)8(10)4-9(13)14-11/h4,7,12H,5-6H2,1-3H3/t7-,11+/m0/s1 | |
Source | PubChem | |
URL | https://pubchem.ncbi.nlm.nih.gov | |
Description | Data deposited in or computed by PubChem | |
InChI Key |
XEVQXKKKAVVSMW-WRWORJQWSA-N | |
Source | PubChem | |
URL | https://pubchem.ncbi.nlm.nih.gov | |
Description | Data deposited in or computed by PubChem | |
Canonical SMILES |
CC1(CC(CC2(C1=CC(=O)O2)C)O)C | |
Source | PubChem | |
URL | https://pubchem.ncbi.nlm.nih.gov | |
Description | Data deposited in or computed by PubChem | |
Isomeric SMILES |
C[C@@]12C[C@H](CC(C1=CC(=O)O2)(C)C)O | |
Source | PubChem | |
URL | https://pubchem.ncbi.nlm.nih.gov | |
Description | Data deposited in or computed by PubChem | |
Molecular Formula |
C11H16O3 | |
Source | PubChem | |
URL | https://pubchem.ncbi.nlm.nih.gov | |
Description | Data deposited in or computed by PubChem | |
DSSTOX Substance ID |
DTXSID501019112 | |
Record name | Loliolide | |
Source | EPA DSSTox | |
URL | https://comptox.epa.gov/dashboard/DTXSID501019112 | |
Description | DSSTox provides a high quality public chemistry resource for supporting improved predictive toxicology. | |
Molecular Weight |
196.24 g/mol | |
Source | PubChem | |
URL | https://pubchem.ncbi.nlm.nih.gov | |
Description | Data deposited in or computed by PubChem | |
CAS No. |
5989-02-6 | |
Record name | Loliolide | |
Source | CAS Common Chemistry | |
URL | https://commonchemistry.cas.org/detail?cas_rn=5989-02-6 | |
Description | CAS Common Chemistry is an open community resource for accessing chemical information. Nearly 500,000 chemical substances from CAS REGISTRY cover areas of community interest, including common and frequently regulated chemicals, and those relevant to high school and undergraduate chemistry classes. This chemical information, curated by our expert scientists, is provided in alignment with our mission as a division of the American Chemical Society. | |
Explanation | The data from CAS Common Chemistry is provided under a CC-BY-NC 4.0 license, unless otherwise stated. | |
Record name | Loliolide | |
Source | ChemIDplus | |
URL | https://pubchem.ncbi.nlm.nih.gov/substance/?source=chemidplus&sourceid=0005989026 | |
Description | ChemIDplus is a free, web search system that provides access to the structure and nomenclature authority files used for the identification of chemical substances cited in National Library of Medicine (NLM) databases, including the TOXNET system. | |
Record name | Loliolide | |
Source | EPA DSSTox | |
URL | https://comptox.epa.gov/dashboard/DTXSID501019112 | |
Description | DSSTox provides a high quality public chemistry resource for supporting improved predictive toxicology. | |
Record name | LOLIOLIDE | |
Source | FDA Global Substance Registration System (GSRS) | |
URL | https://gsrs.ncats.nih.gov/ginas/app/beta/substances/U3BB4IM281 | |
Description | The FDA Global Substance Registration System (GSRS) enables the efficient and accurate exchange of information on what substances are in regulated products. Instead of relying on names, which vary across regulatory domains, countries, and regions, the GSRS knowledge base makes it possible for substances to be defined by standardized, scientific descriptions. | |
Explanation | Unless otherwise noted, the contents of the FDA website (www.fda.gov), both text and graphics, are not copyrighted. They are in the public domain and may be republished, reprinted and otherwise used freely by anyone without the need to obtain permission from FDA. Credit to the U.S. Food and Drug Administration as the source is appreciated but not required. | |
Retrosynthesis Analysis
AI-Powered Synthesis Planning: Our tool employs the Template_relevance Pistachio, Template_relevance Bkms_metabolic, Template_relevance Pistachio_ringbreaker, Template_relevance Reaxys, Template_relevance Reaxys_biocatalysis model, leveraging a vast database of chemical reactions to predict feasible synthetic routes.
One-Step Synthesis Focus: Specifically designed for one-step synthesis, it provides concise and direct routes for your target compounds, streamlining the synthesis process.
Accurate Predictions: Utilizing the extensive PISTACHIO, BKMS_METABOLIC, PISTACHIO_RINGBREAKER, REAXYS, REAXYS_BIOCATALYSIS database, our tool offers high-accuracy predictions, reflecting the latest in chemical research and data.
Strategy Settings
Precursor scoring | Relevance Heuristic |
---|---|
Min. plausibility | 0.01 |
Model | Template_relevance |
Template Set | Pistachio/Bkms_metabolic/Pistachio_ringbreaker/Reaxys/Reaxys_biocatalysis |
Top-N result to add to graph | 6 |
Feasible Synthetic Routes
A: (–)-Loliolide acts as a general signal of plant stress, triggering defensive metabolite responses to competitors, herbivores, and pathogens. [] It induces the production of various defensive compounds, including phenolic acids, flavonoids, terpenoids, alkaloids, benzoxazinoids, and cyanogenic glycosides, irrespective of the plant species. [] This induction is mediated through pathways involving jasmonic acid, hydrogen peroxide, and Ca2+. [, ]
A: Yes, research suggests that (–)-Loliolide can influence both aboveground and belowground plant processes. For instance, it has been shown to delay flowering in tobacco by upregulating flowering suppressors (FT3 and FLC) and transiently downregulating flowering stimulators (AP1 and SOC1). [] Additionally, (–)-Loliolide plays a role in shaping root placement patterns in allelopathic plant-plant interactions, influencing root growth and placement in both emitting and receiving plants. []
ANone: (–)-Loliolide exhibits a range of effects on human cells, including:
- Anti-adipogenic activity: Inhibits adipogenesis in human bone marrow-derived mesenchymal stromal cells by activating AMPK and Wnt/β-catenin pathways. []
- Anti-apoptotic and wound-healing effects: Protects human keratinocytes against apoptosis induced by ultraviolet radiation and enhances the expression of epidermal growth factor receptor signaling pathway (PI3K, AKT) and migration factors, suggesting wound-healing potential. []
- Anti-inflammatory effects: Suppresses oxidative stress and inflammation in IFN-γ/TNF-α-stimulated HaCaT keratinocytes by activating the Nrf2/HO-1 signaling pathway. [, ]
A: (–)-Loliolide (also known as calendin) has a molecular formula of C10H16O3 and a molecular weight of 184.23 g/mol. [, ]
A: Yes, (–)-Loliolide has been extensively studied using various spectroscopic techniques, including UV, IR, 1H-NMR, 13C-NMR, and mass spectrometry. These techniques have been instrumental in confirming its structure and identifying it in various plant and algal species. [, , , , , ]
A: Yes, in silico studies have been conducted to predict the potential mechanisms of action and pharmacological properties of (–)-Loliolide. For example, molecular docking studies have explored its interaction with enzymes involved in the inflammatory cascade, such as cyclooxygenase (COX-1 & 2) and 5-lipooxygenase (5-LOX), suggesting potential as an anti-inflammatory agent. []
ANone: Researchers have employed a variety of in vitro assays to investigate the biological activities of (–)-Loliolide, including:
- MTT assay: To assess cell viability and proliferation in various cell lines, including human keratinocytes (HaCaT), mouse melanoma (B16F10), human bone marrow-derived mesenchymal stromal cells (hBM-MSCs), and hepatocellular carcinoma (HepG2) cells. [, , , , ]
- Transactivation assay: To evaluate the phytoestrogenic activity of (–)-Loliolide and its potential to interact with estrogen receptors α and β. []
ANone: In vivo studies using animal models have demonstrated the following effects of (–)-Loliolide:
- Anti-inflammatory activity: Alleviates carrageenan-induced rat paw edema, suggesting potential as an anti-inflammatory agent. []
- Protection against fine dust-induced oxidative stress: Protects against fine dust-induced oxidative stress in human keratinocytes. []
ANone: While (–)-Loliolide generally exhibits low toxicity in in vitro studies, comprehensive toxicological data are limited. Further investigation is required to determine its safety profile, potential adverse effects, and long-term effects in vivo.
A: (–)-Loliolide was first isolated and identified as a bitter principle from the flowers of Calendula officinalis L. in 1961. [] It was initially named "calendin" but later recognized as (–)-Loliolide due to its presence in various other plant species. [] Key milestones in (–)-Loliolide research include:
- Identification in diverse organisms: Its presence has been confirmed in various plants, algae, and even insects, highlighting its wide distribution in nature. [, ]
- Elucidation of its biosynthesis: Research suggests that (–)-Loliolide is likely derived from carotenoids through oxidative degradation pathways. [, ]
- Discovery of its diverse biological activities: (–)-Loliolide has been shown to possess a wide range of activities, including anti-inflammatory, antioxidant, anti-adipogenic, and plant defense-inducing properties. [, , , , ]
ANone: The diverse biological activities of (–)-Loliolide hold promise for applications in various fields, including:
- Medicine and Pharmacology: Its anti-inflammatory, antioxidant, and potential anticancer activities make it an attractive candidate for developing new therapeutics. [, , ]
- Cosmetics: Its anti-apoptotic, wound-healing, and anti-melanogenic properties suggest potential applications in skincare and cosmetic products. [, ]
- Agriculture: Its role as a plant defense-inducing agent and potential herbicidal activity could be exploited for developing sustainable agricultural practices. [, , , ]
試験管内研究製品の免責事項と情報
BenchChemで提示されるすべての記事および製品情報は、情報提供を目的としています。BenchChemで購入可能な製品は、生体外研究のために特別に設計されています。生体外研究は、ラテン語の "in glass" に由来し、生物体の外で行われる実験を指します。これらの製品は医薬品または薬として分類されておらず、FDAから任何の医療状態、病気、または疾患の予防、治療、または治癒のために承認されていません。これらの製品を人間または動物に体内に導入する形態は、法律により厳格に禁止されています。これらのガイドラインに従うことは、研究と実験において法的および倫理的な基準の遵守を確実にするために重要です。