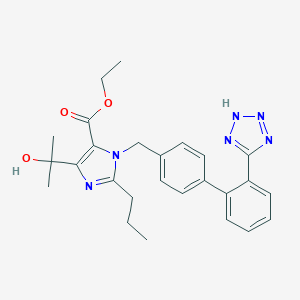
オルメサルタンエチルエステル
説明
Olmesartan is a medication used primarily to treat high blood pressure, and its modification into various ester prodrugs has been a subject of research to improve its pharmacokinetic properties. The ester derivatives of olmesartan, such as the ethyl ester, are designed to enhance the drug's oral bioavailability and stability, making it more effective for patients.
Synthesis Analysis
The synthesis of olmesartan ester prodrugs involves several steps, including condensation, oxidation, esterification, and the Grignard reaction. For instance, the synthesis of ethyl 4-[(1-hydroxy-1-methyl)ethyl]-2-propyl-1H-imidazole-5-carboxylate, a key intermediate of Olmesartan, was achieved with an overall yield of 32.2% using microwave irradiation, which is a novel approach to traditional synthesis methods .
Molecular Structure Analysis
The molecular structure of olmesartan ester prodrugs is characterized by the presence of ester groups that are intended to be cleaved in vivo to release the active olmesartan. The ester derivative IIa, for example, has a n-octyl substituted dioxolone moiety, which is rapidly converted into olmesartan within 30 minutes in rat plasma .
Chemical Reactions Analysis
The ester prodrugs of olmesartan undergo various chemical reactions in the body to release the active drug. For example, olmesartan hexetil showed high stability in simulated gastric juice but was rapidly hydrolyzed to olmesartan in rat liver microsomes and rat plasma . Similarly, the dissolution of olmesartan in methanol generates a highly bioactive methyl ether analogue through an SN1 mechanism involving an intramolecular proton transfer .
Physical and Chemical Properties Analysis
The physical and chemical properties of olmesartan ester prodrugs are tailored to improve the drug's performance. The ester derivative IIa, for instance, showed promising pharmacokinetic parameters when compared with olmesartan medoxomil, suggesting that it could be a more effective prodrug . The olmesartan hexetil prodrug also demonstrated significantly increased C(max) and AUC(last) for olmesartan, indicating a marked increase in oral bioavailability .
科学的研究の応用
合成と物理化学的特性
オルメサルタンメドキソミル (OM) のマルチグラムスケール合成のプロセス開発中に、最終的な有効医薬品成分 (API) と共に、2 つの主要な位置異性体プロセス関連不純物が観察されました。 これらの不純物は、OM の N-1- および N-2- (5-メチル-2-オキソ-1,3-ジオキソレン-4-イル)メチル誘導体として同定されました .
サルタン合成における役割
OM 合成における主要な中間体である N-トリフェニルメチルオルメサルタンエチル (N-トリチルオルメサルタンエチル) および N-トリチルオルメサルタンメドキソミルの分子構造は、単結晶 X 線回折 (SCXRD) を使用して解明および精密化されました。 SCXRD 研究により、OM の N-トリチル化中間体は、2 つの可能な位置異性体のうちの 1 つとしてのみ存在することが明らかになりました .
錠剤の製剤と評価
オルメサルタンエチルエステルは、直接圧縮法によって調製された錠剤の設計、開発、および特性評価に使用されてきました。 主な目的は、有効成分の高溶解速度を確保することでした .
賦形剤との適合性
走査型電子顕微鏡 (SEM)、示差走査熱量測定 (DSC)、およびフーリエ変換赤外分光法 (FT-IR) 研究により、賦形剤の物理的および化学的適合性を有効成分と確実にするために、賦形剤の厳密な選択が行われました .
直接圧縮における役割
SeDeM メソッドを使用して、混合物の直接圧縮における適合性を評価しました。 錠剤は、含量均一性、破砕強度、脆性、および崩壊時間の薬局方仕様を満たしました .
高血圧治療における役割
オルメサルタンは、他の ARA-II よりも AT1 受容体への親和性が高く結合するため、強力で持続的な遮断を保証する高強度サルタンとして選択されました .
作用機序
Target of Action
Olmesartan Ethyl Ester, a prodrug of Olmesartan, primarily targets the Angiotensin II Receptor Type 1 (AT1) . The AT1 receptor plays a crucial role in the Renin-Angiotensin-Aldosterone System (RAAS), which is involved in the regulation of blood pressure and fluid balance .
Mode of Action
As a selective and competitive, nonpeptide angiotensin II receptor antagonist, Olmesartan blocks the vasoconstrictor and aldosterone-secreting effects of angiotensin II . It interacts reversibly at the AT1 and AT2 receptors of many tissues and has slow dissociation kinetics . Its affinity for the AT1 receptor is 12,500 times greater than the AT2 receptor .
Pharmacokinetics
Olmesartan Ethyl Ester is hydrolyzed in the gastrointestinal tract to active Olmesartan . The pharmacokinetic data of Olmesartan is well described by a two-compartment linear pharmacokinetic model with first-order absorption and an absorption lag-time . The mean values of clearance (CL/F) and volume of distribution (V/F) of Olmesartan in patients were 0.31565 L/h and 44.5162 L, respectively . Age and creatinine clearance were factors influencing the clearance of Olmesartan, and the volume of distribution of Olmesartan was dependent on age and body surface area .
Action Environment
The absorption of Olmesartan Ethyl Ester is influenced by the pH of the environment. It is predominantly anionic at intestinal pH, which contributes to its enhanced intestinal absorption . Certain environmental factors such as the presence of OATP2B1 inhibitors can decrease the intestinal absorption of Olmesartan Ethyl Ester . For instance, oral administration of Olmesartan Ethyl Ester with 50 µM naringin to rats significantly reduced the area under the plasma concentration–time curve of Olmesartan to 76.9% .
将来の方向性
Olmesartan, the active metabolite of Olmesartan Ethyl Ester, is commonly used for the management of hypertension and Type 2 Diabetes-associated nephropathy, particularly in patients who are unable to tolerate ACE inhibitors . ARBs such as olmesartan have been shown in a number of large-scale clinical outcomes trials to improve cardiovascular outcomes including reducing risk of myocardial infarction, stroke, the progression of heart failure, and hospitalization . Future research may focus on further understanding the pharmacokinetics and pharmacodynamics of Olmesartan Ethyl Ester, as well as exploring new therapeutic applications.
生化学分析
Biochemical Properties
Olmesartan Ethyl Ester interacts with various enzymes and proteins. It is predominantly anionic at intestinal pH and interacts with the human organic anion transporting polypeptide 2B1 (OATP2B1) expressed in the small intestine .
Cellular Effects
Olmesartan Ethyl Ester influences cell function by interacting with the angiotensin II receptor (AT1R), which is involved in blood pressure regulation . Its impact on cell signaling pathways, gene expression, and cellular metabolism is significant.
Molecular Mechanism
The molecular mechanism of Olmesartan Ethyl Ester involves binding interactions with biomolecules, enzyme inhibition or activation, and changes in gene expression. It exerts its effects at the molecular level, primarily through its interaction with AT1R .
Temporal Effects in Laboratory Settings
In laboratory settings, the effects of Olmesartan Ethyl Ester change over time. It has been observed that the compound exhibits good stability and degradation properties .
Dosage Effects in Animal Models
The effects of Olmesartan Ethyl Ester vary with different dosages in animal models. High doses of the compound can lead to toxic or adverse effects .
Metabolic Pathways
Olmesartan Ethyl Ester is involved in several metabolic pathways. It interacts with enzymes and cofactors, affecting metabolic flux or metabolite levels .
Transport and Distribution
Olmesartan Ethyl Ester is transported and distributed within cells and tissues. It interacts with transporters or binding proteins, affecting its localization or accumulation .
Subcellular Localization
The subcellular localization of Olmesartan Ethyl Ester affects its activity or function. It is directed to specific compartments or organelles through targeting signals or post-translational modifications .
特性
IUPAC Name |
ethyl 5-(2-hydroxypropan-2-yl)-2-propyl-3-[[4-[2-(2H-tetrazol-5-yl)phenyl]phenyl]methyl]imidazole-4-carboxylate | |
---|---|---|
Source | PubChem | |
URL | https://pubchem.ncbi.nlm.nih.gov | |
Description | Data deposited in or computed by PubChem | |
InChI |
InChI=1S/C26H30N6O3/c1-5-9-21-27-23(26(3,4)34)22(25(33)35-6-2)32(21)16-17-12-14-18(15-13-17)19-10-7-8-11-20(19)24-28-30-31-29-24/h7-8,10-15,34H,5-6,9,16H2,1-4H3,(H,28,29,30,31) | |
Source | PubChem | |
URL | https://pubchem.ncbi.nlm.nih.gov | |
Description | Data deposited in or computed by PubChem | |
InChI Key |
RYYGFMDEVYVZGY-UHFFFAOYSA-N | |
Source | PubChem | |
URL | https://pubchem.ncbi.nlm.nih.gov | |
Description | Data deposited in or computed by PubChem | |
Canonical SMILES |
CCCC1=NC(=C(N1CC2=CC=C(C=C2)C3=CC=CC=C3C4=NNN=N4)C(=O)OCC)C(C)(C)O | |
Source | PubChem | |
URL | https://pubchem.ncbi.nlm.nih.gov | |
Description | Data deposited in or computed by PubChem | |
Molecular Formula |
C26H30N6O3 | |
Source | PubChem | |
URL | https://pubchem.ncbi.nlm.nih.gov | |
Description | Data deposited in or computed by PubChem | |
Molecular Weight |
474.6 g/mol | |
Source | PubChem | |
URL | https://pubchem.ncbi.nlm.nih.gov | |
Description | Data deposited in or computed by PubChem | |
Retrosynthesis Analysis
AI-Powered Synthesis Planning: Our tool employs the Template_relevance Pistachio, Template_relevance Bkms_metabolic, Template_relevance Pistachio_ringbreaker, Template_relevance Reaxys, Template_relevance Reaxys_biocatalysis model, leveraging a vast database of chemical reactions to predict feasible synthetic routes.
One-Step Synthesis Focus: Specifically designed for one-step synthesis, it provides concise and direct routes for your target compounds, streamlining the synthesis process.
Accurate Predictions: Utilizing the extensive PISTACHIO, BKMS_METABOLIC, PISTACHIO_RINGBREAKER, REAXYS, REAXYS_BIOCATALYSIS database, our tool offers high-accuracy predictions, reflecting the latest in chemical research and data.
Strategy Settings
Precursor scoring | Relevance Heuristic |
---|---|
Min. plausibility | 0.01 |
Model | Template_relevance |
Template Set | Pistachio/Bkms_metabolic/Pistachio_ringbreaker/Reaxys/Reaxys_biocatalysis |
Top-N result to add to graph | 6 |
Feasible Synthetic Routes
Q & A
Q1: What makes the synthesis method described in the first research paper advantageous for producing Trityl Olmesartan?
A1: The paper highlights the use of tetrahydrofuran and low-level alcohols like methanol as solvents in the hydrolysis of Trityl Olmesartan Ethyl Ester. [] This specific solvent combination, under anhydrous conditions, minimizes side reactions, leading to a higher purity of the final Trityl Olmesartan product and a more efficient synthesis. []
Q2: The second research paper investigates the solubility of N-Trityl Olmesartan Ethyl Ester. Why is understanding the solubility of this compound in various solvents important for pharmaceutical applications?
A2: Solubility plays a crucial role in pharmaceutical development. Determining the solubility of N-Trityl Olmesartan Ethyl Ester in various organic solvents provides essential data for optimizing the synthesis process, purification steps, and potentially even for developing formulations with improved bioavailability. [] While the abstract doesn't provide specific results, this research likely explored how the compound interacts with different solvents, which is valuable information for large-scale production and formulation design.
試験管内研究製品の免責事項と情報
BenchChemで提示されるすべての記事および製品情報は、情報提供を目的としています。BenchChemで購入可能な製品は、生体外研究のために特別に設計されています。生体外研究は、ラテン語の "in glass" に由来し、生物体の外で行われる実験を指します。これらの製品は医薬品または薬として分類されておらず、FDAから任何の医療状態、病気、または疾患の予防、治療、または治癒のために承認されていません。これらの製品を人間または動物に体内に導入する形態は、法律により厳格に禁止されています。これらのガイドラインに従うことは、研究と実験において法的および倫理的な基準の遵守を確実にするために重要です。