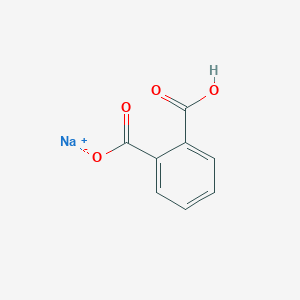
フタル酸水素ナトリウム
- 専門家チームからの見積もりを受け取るには、QUICK INQUIRYをクリックしてください。
- 品質商品を競争力のある価格で提供し、研究に集中できます。
説明
1,2-Benzenedicarboxylic acid, monosodium salt, also known as 1,2-Benzenedicarboxylic acid, monosodium salt, is a useful research compound. Its molecular formula is C8H6NaO4 and its molecular weight is 189.12 g/mol. The purity is usually 95%.
The exact mass of the compound 1,2-Benzenedicarboxylic acid, monosodium salt is unknown and the complexity rating of the compound is unknown. Its Medical Subject Headings (MeSH) category is Chemicals and Drugs Category - Organic Chemicals - Carboxylic Acids - Acids, Carbocyclic - Phthalic Acids - Supplementary Records. The United Nations designated GHS hazard class pictogram is Irritant, and the GHS signal word is WarningThe storage condition is unknown. Please store according to label instructions upon receipt of goods.
BenchChem offers high-quality 1,2-Benzenedicarboxylic acid, monosodium salt suitable for many research applications. Different packaging options are available to accommodate customers' requirements. Please inquire for more information about 1,2-Benzenedicarboxylic acid, monosodium salt including the price, delivery time, and more detailed information at info@benchchem.com.
科学的研究の応用
非線形光学応用
不純物をドープしたフタル酸水素ナトリウムは、第二高調波発生(SHG)効率に影響を与えることが示されています。 これは、光学品質と物理的特性が向上したため、非線形光学(NLO)応用に適した材料になります .
誘電率の向上
フタル酸水素ナトリウム半水和結晶にドーパントを添加すると、誘電率に影響を与える可能性があります。 これは、特定の誘電率を持つ材料を必要とするアプリケーションにとって重要です .
レーザー損傷閾値の向上
フタル酸水素ナトリウム半水和結晶にドーパントを添加すると、レーザー損傷閾値を向上させることもできます。これは、高出力レーザーアプリケーションで使用される材料にとって重要です .
強誘電性
フタル酸水素ナトリウム結晶は、ドープした場合、強誘電性を示すことが報告されています。これは、メモリ記憶装置や電子スイッチに役立つ可能性があります .
フォトルミネッセンス特性
フタル酸水素ナトリウムのフォトルミネッセンス特性は、ドーピングによって影響を受ける可能性があり、さまざまな技術の蛍光材料の作成に役立ちます .
シンチレーション材料の結晶成長
フタル酸水素ナトリウムは、放射線検出や医用画像技術で重要な高エネルギーシンチレーション材料の結晶成長プロセスに使用されています .
作用機序
Target of Action
Sodium hydrogen phthalate, also known as sodium biphthalate or 1,2-Benzenedicarboxylic acid, monosodium salt, is primarily used as a buffer in biological research and as a primary standard for acid-base titrations .
Mode of Action
Sodium hydrogen phthalate acts as a weak acid and can donate a proton (H+) in solution, which is fundamental to its role as a buffer and titration standard . In the context of titrations, it reacts with bases like sodium hydroxide (NaOH) in a neutralization reaction . This interaction allows for the precise determination of the concentration of the base .
Pharmacokinetics
As a small, water-soluble molecule, it is likely to be well-absorbed and distributed throughout the body if ingested or administered .
Result of Action
The primary result of sodium hydrogen phthalate’s action is the maintenance of a stable pH in a solution, which is crucial for many biological and chemical processes . In the context of a titration, its reaction with a base allows for the precise determination of the base’s concentration .
Action Environment
The action of sodium hydrogen phthalate can be influenced by environmental factors such as temperature and the presence of other ions in solution. For example, its solubility and reactivity can change with temperature . Additionally, its effectiveness as a buffer can be influenced by the concentration of other ions in the solution.
生化学分析
Biochemical Properties
Sodium hydrogen phthalate is known to participate in various biochemical reactions . It interacts with enzymes, proteins, and other biomolecules, influencing their function and activity. For instance, it has been reported that sodium hydrogen phthalate can be decomposed into phthalates (DBP) and Diuretic sylycol (DEP), then further to Phthalic acid (PA). PA was oxidized, dehydrogenated, and decarboxylated into protocatechins, further entered the TCA cycle through orthotopic ring opening .
Cellular Effects
The effects of sodium hydrogen phthalate on various types of cells and cellular processes are diverse. It has been reported that phthalates, a group of compounds that includes sodium hydrogen phthalate, can have significant impacts on cell viability and Nrf2 of HepG2 . Furthermore, phthalates can dysregulate the levels and activity of the HPA axis at multiple levels .
Molecular Mechanism
The molecular mechanism of action of sodium hydrogen phthalate involves interactions with biomolecules, enzyme inhibition or activation, and changes in gene expression. The mechanism is one of polar interactions between the polar centers of the phthalate molecule (the C=O functionality) and the positively charged areas of the vinyl chain, typically residing on the carbon atom of the carbon-chlorine bond .
Temporal Effects in Laboratory Settings
In laboratory settings, the effects of sodium hydrogen phthalate can change over time. For instance, it has been observed that as the time of phthalate exposure increased, cell viability gradually decreased .
Dosage Effects in Animal Models
The effects of sodium hydrogen phthalate can vary with different dosages in animal models. For instance, phthalates exhibit low acute toxicity in animal models or humans with LD50 values being between or above 1–30 g/kg body weight .
Metabolic Pathways
Sodium hydrogen phthalate is involved in various metabolic pathways. For instance, the degradation pathway of DEHP, a compound similar to sodium hydrogen phthalate, was analyzed by GC-MS. DEHP was decomposed into phthalates (DBP) and Diuretic sylycol (DEP), then further to Phthalic acid (PA). PA was oxidized, dehydrogenated, and decarboxylated into protocatechins, further entered the TCA cycle through orthotopic ring opening .
Transport and Distribution
Sodium hydrogen phthalate is transported and distributed within cells and tissues. Sodium transporters play a crucial role in this process . They maintain a sodium gradient utilized by multiple sodium-dependent transport mechanisms to regulate various cellular functions .
Subcellular Localization
It is known that sodium transporters, which could potentially interact with sodium hydrogen phthalate, are ubiquitously expressed plasma membrane proteins .
生物活性
1,2-Benzenedicarboxylic acid, monosodium salt, commonly known as sodium hydrogen phthalate, is a dicarboxylic acid derivative widely used in various biological and chemical applications. This article presents a detailed examination of its biological activity, including its mechanisms of action, biochemical properties, and potential therapeutic implications.
- Molecular Formula : C8H5O4Na
- Molecular Weight : 182.12 g/mol
- Structure : Comprises a benzene ring with two carboxyl groups (-COOH) at the 1 and 2 positions, with one of the carboxyl groups ionized as a sodium salt.
Sodium hydrogen phthalate functions primarily as a buffer in biological systems. Its ability to donate protons (H+) makes it essential for maintaining pH stability in various biochemical reactions. This property is crucial in laboratory settings, particularly for titrations and enzyme assays where pH fluctuations can significantly affect outcomes.
Interaction with Biomolecules
Research indicates that sodium hydrogen phthalate interacts with various biomolecules, including enzymes and proteins. These interactions can influence enzymatic activity and cellular processes. For instance, studies have shown that it can modulate the activity of certain enzymes involved in metabolic pathways .
Cellular Effects
The compound has been observed to affect cell viability and proliferation. Notably, it can induce oxidative stress in cell cultures, leading to alterations in gene expression and cellular function. In HepG2 cells (a human liver cancer cell line), sodium hydrogen phthalate has been shown to inhibit cell growth by affecting the Nrf2 pathway, which is crucial for cellular defense against oxidative damage .
Antioxidant Activity
A study investigated the antioxidant properties of sodium hydrogen phthalate using various assays. The results indicated that it possesses significant free radical scavenging ability, which may contribute to its protective effects against oxidative stress in biological systems .
Assay Type | IC50 Value (μg/mL) |
---|---|
DPPH Scavenging Activity | 150 ± 5 |
β-Carotene-Linoleic Bleaching | 200 ± 10 |
Cytotoxicity Studies
In vitro cytotoxicity assessments have demonstrated that sodium hydrogen phthalate exhibits varying degrees of toxicity depending on concentration and exposure duration. For example, at higher concentrations (≥100 μg/mL), significant cytotoxic effects were observed in human-derived cell lines .
Concentration (μg/mL) | Cell Viability (%) |
---|---|
0 | 100 |
50 | 85 |
100 | 60 |
200 | 30 |
Potential Therapeutic Applications
Given its biochemical properties and interactions with cellular mechanisms, sodium hydrogen phthalate may have potential therapeutic applications:
- Antioxidant Therapy : Its antioxidant capabilities suggest it could be beneficial in conditions characterized by oxidative stress.
- Buffering Agent : Its role as a buffering agent is crucial in pharmaceutical formulations where pH control is necessary.
- Research Applications : Used extensively as a primary standard in acid-base titrations due to its stability and predictable behavior in solution.
特性
CAS番号 |
827-27-0 |
---|---|
分子式 |
C8H6NaO4 |
分子量 |
189.12 g/mol |
IUPAC名 |
sodium;2-carboxybenzoate |
InChI |
InChI=1S/C8H6O4.Na/c9-7(10)5-3-1-2-4-6(5)8(11)12;/h1-4H,(H,9,10)(H,11,12); |
InChIキー |
DFLCZDIXTAAMLZ-UHFFFAOYSA-N |
SMILES |
C1=CC=C(C(=C1)C(=O)O)C(=O)[O-].[Na+] |
異性体SMILES |
C1=CC=C(C(=C1)C(=O)O)C(=O)[O-].[Na+] |
正規SMILES |
C1=CC=C(C(=C1)C(=O)O)C(=O)O.[Na] |
Key on ui other cas no. |
10197-71-4 827-27-0 |
ピクトグラム |
Irritant |
関連するCAS |
88-99-3 (Parent) |
同義語 |
disodium phthalate phthalate phthalic acid phthalic acid, copper salt phthalic acid, dipotassium salt phthalic acid, disodium salt phthalic acid, monobarium salt phthalic acid, monocalcium salt phthalic acid, monoiron (2+) salt phthalic acid, monolead (2+) salt phthalic acid, monopotassium salt phthalic acid, monoruthenium salt phthalic acid, monosodium salt phthalic acid, potassium salt phthalic acid, potassium, sodium salt phthalic acid, sodium salt potassium hydrogen phthalate |
製品の起源 |
United States |
Q1: How is sodium hydrogen phthalate used in analytical chemistry?
A: Sodium hydrogen phthalate serves as a versatile reagent in analytical techniques like ion chromatography. Research shows its effectiveness in separating inorganic anions such as iodate, phosphate, and nitrate. [] This separation is achieved through a reversed-phase high-performance liquid chromatography (HPLC) setup where a stationary phase is conditioned with hexadecyltrimethylammonium bromide (CTAB) and sodium hydrogen phthalate. The varying interactions of different anions with this modified stationary phase allow for their effective separation and subsequent detection via indirect ultraviolet absorption. []
Q2: What unique properties make sodium hydrogen phthalate suitable for crystal doping?
A: Sodium hydrogen phthalate hemihydrate (SP) crystals, when doped with metal ions like nickel (Ni2+) or iron (Fe3+), exhibit enhanced optical and thermal properties. [, ] These doped crystals show improved transparency in the UV-Vis region and higher thermal stability compared to pure SP crystals. This makes them promising for applications requiring specific optical properties and thermal resilience. [, ]
Q3: Can sodium hydrogen phthalate influence the self-assembly of surfactant systems?
A: Yes, sodium hydrogen phthalate (SHP) can significantly influence the self-assembly behavior of cationic surfactant solutions. Studies using the surfactant 3-hexadecyloxy-2-hydroxypropyltrimethylammonium bromide (R16HTAB) demonstrate that the addition of SHP induces the formation of wormlike micelles. [] This change in micellar structure is attributed to the unique binding interactions between SHP and the surfactant molecules. Furthermore, this mixed system exhibits remarkable pH and temperature responsiveness, transitioning between a water-like and gel-like state depending on these environmental factors. [] This dual-responsive behavior is attributed to the dynamic nature of SHP-surfactant interactions under varying conditions, making it a promising system for stimuli-responsive material applications. []
Q4: How is sodium hydrogen phthalate characterized structurally?
A: Sodium hydrogen phthalate can be characterized using various spectroscopic techniques. Fourier transform infrared (FTIR) and Raman spectroscopy help in identifying the vibrational modes of the molecule and deducing its structure. [] X-ray diffraction (XRD) provides insights into its crystal structure, revealing an orthorhombic phase for both pure and metal-doped sodium hydrogen phthalate hemihydrate crystals. []
試験管内研究製品の免責事項と情報
BenchChemで提示されるすべての記事および製品情報は、情報提供を目的としています。BenchChemで購入可能な製品は、生体外研究のために特別に設計されています。生体外研究は、ラテン語の "in glass" に由来し、生物体の外で行われる実験を指します。これらの製品は医薬品または薬として分類されておらず、FDAから任何の医療状態、病気、または疾患の予防、治療、または治癒のために承認されていません。これらの製品を人間または動物に体内に導入する形態は、法律により厳格に禁止されています。これらのガイドラインに従うことは、研究と実験において法的および倫理的な基準の遵守を確実にするために重要です。