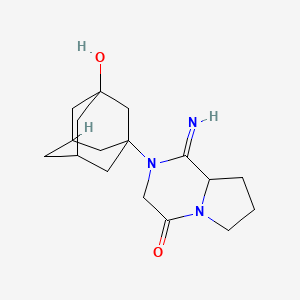
Vildagliptin Impurity E
概要
説明
Vildagliptin Impurity E is a byproduct formed during the synthesis of Vildagliptin, a dipeptidyl peptidase-4 inhibitor used in the treatment of type 2 diabetes mellitus. The control and evaluation of impurities in pharmaceutical compounds are crucial to ensure the quality, safety, and efficacy of the drug .
作用機序
Target of Action
Vildagliptin, the parent compound of Vildagliptin Impurity E, is a member of a new class of hypoglycemic drugs known as dipeptidylpeptidase-4 (DPP-4) inhibitors . The primary targets of Vildagliptin are the pancreatic α and β cells . By selectively inhibiting DPP-4, Vildagliptin increases the glucose sensitivity of these cells .
Mode of Action
Vildagliptin’s mode of action involves the inhibition of the enzyme type 4 dipeptidylpeptidase (DPP-4). This inhibition increases the level and activity of incretins . Incretins are gastrointestinal tract hormones that regulate glucose homeostasis . By increasing the insulin: glucagon ratio, Vildagliptin decreases hepatic glucose production, which leads to a decrease in the plasma glucose level .
Biochemical Pathways
The biochemical pathways affected by Vildagliptin involve the regulation of glucose homeostasis using gastrointestinal tract hormones, i.e., incretins . Two glucagon-like peptides, GLP-1 and GLP-2, were identified as incretins . Incretins undergo rapid cleavage by the enzyme type 4 dipeptidylpeptidase (DPP-4), which decreases their insulinotropic effects . Inhibition of dpp-4 by vildagliptin increases the level and activity of incretins .
Pharmacokinetics
Vildagliptin possesses several desirable pharmacokinetic properties. Following oral administration, Vildagliptin is rapidly and well absorbed with an absolute bioavailability of 85% . The elimination half-life after oral administration is approximately three hours .
Result of Action
The molecular and cellular effects of Vildagliptin’s action involve an increase in the insulin secretory tone . This means that Vildagliptin improves β-cell function by increasing insulin secretion at any given glucose level . This has important clinical implications regarding the effects of Vildagliptin in both fasting and fed states .
Action Environment
The action environment of Vildagliptin can be influenced by various factors. For instance, the presence of impurities might increase the cytotoxicity and oxidative stress of the pharmaceutical formulations at the concentrations studied . Furthermore, the hydrolysis of Vildagliptin is believed to take place in the kidney, resulting in the formation of LAY 151 .
生化学分析
Biochemical Properties
Vildagliptin Impurity E plays a role in biochemical reactions by interacting with various enzymes, proteins, and other biomolecules. It is known to inhibit the enzyme dipeptidyl peptidase-4 (DPP-4), similar to vildagliptin. This inhibition increases the levels of incretin hormones, which in turn enhances insulin secretion and decreases glucagon release. The interaction between this compound and DPP-4 is crucial for its biochemical activity, as it prevents the degradation of incretin hormones, thereby improving glucose homeostasis .
Cellular Effects
This compound influences various cellular processes, including cell signaling pathways, gene expression, and cellular metabolism. By inhibiting DPP-4, this compound increases the levels of incretin hormones, which activate signaling pathways that enhance insulin secretion from pancreatic beta cells. This leads to improved glucose uptake by cells and a reduction in blood glucose levels. Additionally, this compound may affect gene expression related to glucose metabolism and insulin signaling, further contributing to its hypoglycemic effects .
Molecular Mechanism
The molecular mechanism of this compound involves its binding to the active site of DPP-4, thereby inhibiting its enzymatic activity. This inhibition prevents the cleavage of incretin hormones, such as glucagon-like peptide-1 (GLP-1) and glucose-dependent insulinotropic polypeptide (GIP). As a result, the levels of these hormones increase, leading to enhanced insulin secretion and decreased glucagon release. This compound may also influence gene expression by modulating transcription factors involved in glucose metabolism .
Temporal Effects in Laboratory Settings
In laboratory settings, the effects of this compound may change over time due to its stability and degradation. Studies have shown that this compound is relatively stable under standard laboratory conditions, but it may degrade over extended periods or under specific conditions, such as high temperatures or exposure to light. Long-term effects on cellular function have been observed in in vitro and in vivo studies, indicating that prolonged exposure to this compound can lead to sustained improvements in glucose homeostasis .
Dosage Effects in Animal Models
The effects of this compound vary with different dosages in animal models. At low doses, this compound effectively inhibits DPP-4 and improves glucose homeostasis without causing significant adverse effects. At higher doses, toxic effects may be observed, including liver and kidney damage. Threshold effects have been noted, where the efficacy of this compound plateaus beyond a certain dosage, indicating that increasing the dose further does not enhance its hypoglycemic effects .
Metabolic Pathways
This compound is involved in metabolic pathways related to glucose metabolism. By inhibiting DPP-4, it increases the levels of incretin hormones, which enhance insulin secretion and decrease glucagon release. This leads to improved glucose uptake by cells and a reduction in blood glucose levels. This compound may also interact with other enzymes and cofactors involved in glucose metabolism, further contributing to its hypoglycemic effects .
Transport and Distribution
Within cells and tissues, this compound is transported and distributed through various mechanisms. It may interact with transporters and binding proteins that facilitate its uptake and distribution. The localization and accumulation of this compound within specific tissues, such as the pancreas and liver, are crucial for its hypoglycemic effects. These interactions ensure that this compound reaches its target sites and exerts its biochemical activity .
Subcellular Localization
The subcellular localization of this compound is essential for its activity and function. It may be directed to specific compartments or organelles through targeting signals or post-translational modifications. For example, this compound may localize to the endoplasmic reticulum or Golgi apparatus, where it can interact with DPP-4 and other biomolecules involved in glucose metabolism. These interactions are crucial for the inhibition of DPP-4 and the subsequent increase in incretin hormone levels .
準備方法
The synthesis of Vildagliptin and its impurities, including Vildagliptin Impurity E, involves several steps. The primary synthetic route for Vildagliptin uses L-prolinamide and 3-aminoadamantanol as starting materials . The reaction conditions typically involve the use of solvents, catalysts, and controlled temperatures to facilitate the formation of the desired product and its impurities. Industrial production methods often employ high-performance liquid chromatography to separate and purify the final product from its impurities .
化学反応の分析
Vildagliptin Impurity E undergoes various chemical reactions, including oxidation, reduction, and substitution reactions. Common reagents used in these reactions include oxidizing agents, reducing agents, and nucleophiles. The major products formed from these reactions depend on the specific conditions and reagents used. For instance, oxidation reactions may lead to the formation of hydroxylated derivatives, while substitution reactions can result in the replacement of functional groups .
科学的研究の応用
Vildagliptin Impurity E is primarily studied in the context of pharmaceutical research to understand its formation, stability, and potential impact on the efficacy and safety of Vildagliptin. Analytical methods such as ultra-performance liquid chromatography and mass spectrometry are used to detect and quantify this impurity in drug formulations . Research on this compound also contributes to the development of improved synthetic routes and purification techniques to minimize its presence in the final product .
類似化合物との比較
Vildagliptin Impurity E can be compared with other impurities formed during the synthesis of Vildagliptin, such as L-prolinamide and 3-aminoadamantanol derivatives . These impurities share similar synthetic origins but differ in their chemical structures and properties. The uniqueness of this compound lies in its specific formation pathway and the potential impact it may have on the final drug product .
Similar compounds include:
- L-prolinamide derivatives
- 3-aminoadamantanol derivatives
- Other byproducts formed during the synthesis of dipeptidyl peptidase-4 inhibitors .
特性
IUPAC Name |
2-(3-hydroxy-1-adamantyl)-1-imino-6,7,8,8a-tetrahydro-3H-pyrrolo[1,2-a]pyrazin-4-one | |
---|---|---|
Source | PubChem | |
URL | https://pubchem.ncbi.nlm.nih.gov | |
Description | Data deposited in or computed by PubChem | |
InChI |
InChI=1S/C17H25N3O2/c18-15-13-2-1-3-19(13)14(21)9-20(15)16-5-11-4-12(6-16)8-17(22,7-11)10-16/h11-13,18,22H,1-10H2 | |
Source | PubChem | |
URL | https://pubchem.ncbi.nlm.nih.gov | |
Description | Data deposited in or computed by PubChem | |
InChI Key |
ZZYCWDSBZNHPIN-UHFFFAOYSA-N | |
Source | PubChem | |
URL | https://pubchem.ncbi.nlm.nih.gov | |
Description | Data deposited in or computed by PubChem | |
Canonical SMILES |
C1CC2C(=N)N(CC(=O)N2C1)C34CC5CC(C3)CC(C5)(C4)O | |
Source | PubChem | |
URL | https://pubchem.ncbi.nlm.nih.gov | |
Description | Data deposited in or computed by PubChem | |
Molecular Formula |
C17H25N3O2 | |
Source | PubChem | |
URL | https://pubchem.ncbi.nlm.nih.gov | |
Description | Data deposited in or computed by PubChem | |
Molecular Weight |
303.4 g/mol | |
Source | PubChem | |
URL | https://pubchem.ncbi.nlm.nih.gov | |
Description | Data deposited in or computed by PubChem | |
Retrosynthesis Analysis
AI-Powered Synthesis Planning: Our tool employs the Template_relevance Pistachio, Template_relevance Bkms_metabolic, Template_relevance Pistachio_ringbreaker, Template_relevance Reaxys, Template_relevance Reaxys_biocatalysis model, leveraging a vast database of chemical reactions to predict feasible synthetic routes.
One-Step Synthesis Focus: Specifically designed for one-step synthesis, it provides concise and direct routes for your target compounds, streamlining the synthesis process.
Accurate Predictions: Utilizing the extensive PISTACHIO, BKMS_METABOLIC, PISTACHIO_RINGBREAKER, REAXYS, REAXYS_BIOCATALYSIS database, our tool offers high-accuracy predictions, reflecting the latest in chemical research and data.
Strategy Settings
Precursor scoring | Relevance Heuristic |
---|---|
Min. plausibility | 0.01 |
Model | Template_relevance |
Template Set | Pistachio/Bkms_metabolic/Pistachio_ringbreaker/Reaxys/Reaxys_biocatalysis |
Top-N result to add to graph | 6 |
Feasible Synthetic Routes
試験管内研究製品の免責事項と情報
BenchChemで提示されるすべての記事および製品情報は、情報提供を目的としています。BenchChemで購入可能な製品は、生体外研究のために特別に設計されています。生体外研究は、ラテン語の "in glass" に由来し、生物体の外で行われる実験を指します。これらの製品は医薬品または薬として分類されておらず、FDAから任何の医療状態、病気、または疾患の予防、治療、または治癒のために承認されていません。これらの製品を人間または動物に体内に導入する形態は、法律により厳格に禁止されています。これらのガイドラインに従うことは、研究と実験において法的および倫理的な基準の遵守を確実にするために重要です。