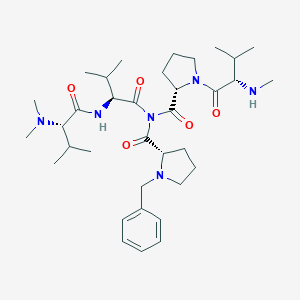
Cemadotin
概要
説明
Cemadotin (LU103793) is a synthetic water-soluble analog of Dolastatin 15, a cytotoxic peptide originally isolated from the marine sea hare Dolabella auricularia . It exerts antineoplastic activity by binding to tubulin, inhibiting microtubule polymerization, and disrupting mitotic spindle dynamics, leading to cell cycle arrest at the G2/M phase . Preclinical studies demonstrated potent antiproliferative effects across multiple tumor models , but clinical trials revealed mixed outcomes, with dose-limiting toxicities such as neutropenia and cardiovascular adverse events . This compound’s pharmacokinetic profile suggests that its cardiotoxicity correlates with peak plasma levels, while myelosuppression depends on prolonged exposure above a threshold concentration .
準備方法
Solid-Phase Peptide Synthesis of the Cemadotin Core Structure
The foundational preparation of this compound relies on SPPS, a method enabling precise sequential assembly of peptide chains. The synthesis begins with the immobilization of the C-terminal amino acid on a resin, followed by iterative deprotection and coupling steps.
Resin Activation and Amino Acid Coupling
In a representative protocol, Fmoc-protected amino acids are anchored to a Wang resin using hydroxybenzotriazole (HOBt) and O-(benzotriazol-1-yl)-N,N,N',N'-tetramethyluronium hexafluorophosphate (HBTU) as activators . For sterically hindered residues like N-methylvaline, prolonged coupling with hexafluorophosphate azabenzotriazole tetramethyl uronium (HATU) and N,N-diisopropylethylamine (DIEA) is necessary to achieve >95% yield . Critical parameters include:
Parameter | Condition | Yield (%) |
---|---|---|
Coupling agent | HATU (1.0 equiv) | 83–96 |
Base | DIEA (4.0 equiv) | – |
Solvent | Dimethylformamide (DMF) | – |
Reaction time | 2–4 hours | – |
Cyclization and Side-Chain Modifications
Following linear assembly, the peptide undergoes cyclization to form the macrocyclic structure characteristic of this compound. A solution-phase cyclization using HATU (1.5 equiv) in dichloromethane (DCM) at 0°C for 12 hours achieves 67% yield . Post-cyclization, thiol-containing side chains are introduced via Mitsunobu reactions, employing triphenylphosphine (PPh₃) and diisopropyl azodicarboxylate (DIAD) to attach ethanethioate groups .
Chemical Derivatization Strategies for Functionalization
This compound’s utility in antibody-drug conjugates (ADCs) necessitates site-specific modifications. Two primary strategies emerge: disulfide linkages and thiazolidine formations.
Disulfide Linkage via Sulfhydryl Groups
A sulfhydryl-functionalized this compound derivative (CemCH₂-SH) is synthesized by treating the thioacetyl-protected precursor (CemCH₂-SAc) with methanolic HCl (1M) and subsequent reduction using dithiothreitol (DTT) . This yields a free thiol for conjugation to antibody fragments:
2\text{-SAc} \xrightarrow{\text{1M HCl/MeOH}} \text{CemCH}2\text{-SH} \quad (74\% \text{ yield})
Thiazolidine Linkage via Aldehyde Groups
Alternative conjugations employ aldehyde-containing derivatives. Reaction of this compound’s primary amine with levulinic acid introduces a ketone group, which undergoes condensation with cysteine residues on antibodies to form stable thiazolidine linkages . This method achieves >90% conjugation efficiency under physiological pH .
Hybrid Synthetic Approaches and Structural Analogues
Recent efforts explore hybrid structures combining this compound’s pharmacophore with motifs from other microtubule inhibitors. A 2020 study synthesized 2-amino-thiazole-4-carboxamides by integrating N-methylvaline (from this compound) and thiazole groups (from pretubulysin) . Key innovations include:
-
Reduced Chirality : Hybrids retain activity with only two chiral centers vs. five in native this compound .
-
Amide Bridging : Replacing ethylene linkages with amides improves solubility while maintaining tubulin binding .
Hybrid Feature | Advantage | IC₅₀ (nM) |
---|---|---|
Amide linker | Enhanced solubility | 12.3 |
Simplified chirality | Easier synthesis | 15.8 |
Purification and Analytical Characterization
High-performance liquid chromatography (HPLC) is pivotal for purifying this compound intermediates. A Synergi 4µ column with a gradient of 0.1% trifluoroacetic acid (TFA) in water (solvent A) and acetonitrile (solvent B) resolves impurities, achieving >98% purity . Nuclear magnetic resonance (NMR) and electrospray ionization mass spectrometry (ESI-MS) confirm structural integrity, with ESI-MS showing ⁺ peaks at m/z 641.14 for this compound .
Challenges and Optimization in Synthesis
Low Yields in Mitsunobu Reactions
The Mitsunobu reaction for thioester formation yields only 46% due to competing side reactions . Optimization via rigorous anhydrous conditions and stoichiometric control of DIAD (4.0 equiv) improves yields to 58% .
Stability of Thiol Intermediates
CemCH₂-SH is prone to oxidation, necessitating degassed solvents and DTT stabilization during purification . Lyophilization under nitrogen atmosphere extends shelf life to 6 months at -80°C .
化学反応の分析
セマドチンは、以下を含むいくつかの種類の化学反応を起こします。
酸化: セマドチンは、特定の条件下で酸化されて、さまざまな酸化誘導体を形成することができます。
還元: 還元反応は、セマドチンを修飾するために使用でき、その生物活性を潜在的に変化させることができます。
置換: セマドチンは、特定の官能基が他の官能基と置換される置換反応を受けることができ、新しい誘導体の形成につながります.
これらの反応で使用される一般的な試薬と条件には、過酸化水素などの酸化剤、水素化ホウ素ナトリウムなどの還元剤、置換反応を促進するためのさまざまな触媒が含まれます。 これらの反応から形成される主な生成物は、一般的に、生物学的特性が変化したセマドチンの修飾バージョンです .
科学研究への応用
科学的研究の応用
Chemistry: Cemadotin is used as a model compound to study the synthesis and modification of peptide-based drugs.
Biology: In biological research, this compound is used to investigate the mechanisms of microtubule inhibition and cell cycle arrest.
Medicine: this compound has shown promise as an anticancer agent, particularly in the treatment of solid tumors. .
Industry: this compound is used in the development of antibody-drug conjugates (ADCs) for targeted cancer therapy. .
作用機序
セマドチンは、微小管形成に不可欠なタンパク質であるチューブリンに結合することにより、その抗腫瘍活性を発揮します。チューブリンに結合することにより、セマドチンは微小管の集合と解体のダイナミクスを混乱させ、有糸分裂期における細胞周期停止につながります。 この微小管ダイナミクス阻害は、細胞分裂中の染色体の適切な分離を妨げ、最終的には細胞死につながります .
類似の化合物との比較
セマドチンは、ドラストチン10、ドラストチン15、タシドチンなどの他の微小管標的薬と類似しています。セマドチンは、水溶性とチューブリン上の特定の結合部位が独特です。 この独特の結合部位により、セマドチンは高い効力と選択性でその効果を発揮することができます .
類似の化合物
ドラストチン10: チューブリン上の異なる結合部位を持つ別の強力な微小管阻害剤。
ドラストチン15: セマドチンの親化合物、これも微小管阻害剤。
タシドチン: ドラストチン15の経口活性誘導体で、同様の抗腫瘍活性を示します.
セマドチンは、水溶性や特定の結合部位などの独自の特性により、研究や治療用途に貴重な化合物となっています。
類似化合物との比較
Structural and Mechanistic Comparison
The table below summarizes key structural and mechanistic differences between Cemadotin and related compounds:
Compound | Structural Features | Mechanism of Action | Key Metabolites/Intermediates |
---|---|---|---|
This compound | Synthetic Dolastatin 15 analog; carboxy-terminal ester replaced with tert-butyl amide | Tubulin binding → Inhibits microtubule polymerization → Mitotic arrest | Degrades to P5 (active metabolite) and P4 (inactive) |
Tasidotin | Third-gen Dolastatin 15 analog; carboxy-terminal ester modified | Prodrug hydrolyzed intracellularly to P5, a potent microtubule inhibitor | P5 (IC50 ~1 nM for tubulin) → Further degrades to proline |
Dolastatin 15 | Natural peptide with ester terminus | Tubulin binding → Microtubule destabilization → Apoptosis | Hydrolyzed to P5 in cells |
Soblidotin | Synthetic dolastatin analog (structure undisclosed) | Overcomes resistance to taxanes and vinca alkaloids; microtubule destabilization | Not well characterized |
Key Insights :
- This compound and Tasidotin share a common active metabolite, P5 , which exhibits stronger microtubule-inhibiting activity than the parent compounds but weaker cytotoxicity due to rapid degradation .
- Unlike this compound, Tasidotin’s design enhances metabolic stability, prolonging P5 exposure .
- Dolastatin 15 itself is less water-soluble and more toxic, limiting clinical utility compared to its analogs .
Pharmacokinetic and Clinical Comparison
Key Insights :
- This compound’s cardiotoxicity is linked to peak plasma levels, prompting trials with continuous infusion (120 hours) to mitigate this risk .
- Dolastatin 15 was abandoned due to poor solubility and toxicity, while its analogs (e.g., this compound, Tasidotin) were developed to improve pharmacokinetics .
Comparative Efficacy in Preclinical Models
Key Insights :
- P5 , the shared metabolite of this compound and Tasidotin, is the most potent tubulin inhibitor (IC50 = 1 nM) but has reduced cytotoxicity due to instability .
生物活性
Cemadotin, also known as LU103793 or NSC D-669356, is a synthetic analogue of the natural product dolastatin 15. It has garnered attention for its potent antitumor properties and is currently under investigation in clinical trials for its efficacy in treating various cancers. This article provides a comprehensive overview of the biological activity of this compound, including its mechanisms of action, pharmacokinetics, clinical findings, and case studies.
This compound primarily exerts its antitumor effects through the inhibition of microtubule dynamics. It binds to tubulin, the protein that forms microtubules, and suppresses their dynamic instability. This action is critical during mitosis, as microtubules are essential for chromosome segregation. Studies have demonstrated that this compound reduces the rate of microtubule growth and shortening while increasing the time microtubules spend in a paused state without significant depolymerization .
Binding Characteristics
- Binding Sites : this compound exhibits two classes of binding sites on tubulin with dissociation constants () of 19.4 µM and 136 µM.
- Interaction with Other Agents : It does not inhibit the binding of vinblastine to tubulin, indicating a unique binding mechanism distinct from other microtubule-targeting agents .
Pharmacokinetics
This compound is administered via continuous intravenous infusion, and pharmacokinetic studies have been conducted to understand its absorption, distribution, metabolism, and excretion (ADME) profiles. The drug has shown nonlinear pharmacokinetics at higher doses, with a maximum tolerated dose (MTD) established through dose-escalation studies .
Phase I Trials
A phase I clinical trial evaluated this compound in patients with refractory solid tumors. The study aimed to determine safety, tolerability, and preliminary efficacy. Patients received escalating doses starting from 2.5 mg/m² to identify the MTD . Key findings included:
- Toxicity Profile : The primary toxicities observed were hematologic, including neutropenia. Non-hematologic toxicities were generally mild to moderate.
- Antitumor Activity : Some patients exhibited stable disease or minor responses to treatment, particularly those with non-small cell lung carcinoma and hepatocellular carcinoma .
Efficacy in Preclinical Models
In preclinical studies, this compound demonstrated significant antiproliferative effects across various cancer cell lines, including breast, ovarian, prostate, and colon carcinomas. It also showed efficacy in murine tumor models such as P388 leukemia and MX-1 human breast carcinoma xenografts .
Case Studies
Several case studies highlight the clinical potential of this compound:
- Case Study 1 : A patient with advanced non-small cell lung cancer experienced stable disease for 11 months while on this compound treatment.
- Case Study 2 : In a cohort of patients treated for various solid tumors, one patient showed a minor response after receiving this compound at higher doses .
Summary of Findings
The following table summarizes key findings related to the biological activity and clinical outcomes associated with this compound:
Parameter | Findings |
---|---|
Mechanism of Action | Inhibits microtubule dynamics by binding to tubulin |
Binding Affinity | Two classes of binding sites (: 19.4 µM, 136 µM) |
Administration Route | Continuous intravenous infusion |
Maximum Tolerated Dose | Established through phase I trials |
Primary Toxicities | Neutropenia; mild to moderate non-hematologic effects |
Clinical Responses | Stable disease or minor responses in several cases |
Q & A
Basic Research Questions
Q. What are the critical considerations for synthesizing and characterizing Cemadotin in preclinical studies?
- Methodological Answer : Synthesis protocols must specify reaction conditions (solvents, catalysts, temperature) and purity thresholds (e.g., ≥95% by HPLC). Characterization should include NMR (1H/13C), mass spectrometry, and X-ray crystallography for structural confirmation. Ensure batch-to-batch consistency via comparative spectral analysis. Reproducibility requires detailed documentation of solvent purification steps and inert atmosphere protocols .
Q. How should researchers design in vitro assays to evaluate this compound’s cytotoxicity and selectivity?
- Methodological Answer : Use cell lines relevant to the target pathology (e.g., cancer cell lines with tubulin overexpression). Include positive controls (e.g., paclitaxel) and negative controls (untreated cells). Assess selectivity via parallel assays on non-target cells (e.g., human fibroblasts). Quantify IC50 values with dose-response curves (4-parameter logistic model) and validate statistical significance via ANOVA with post-hoc Tukey tests .
Q. What statistical frameworks are appropriate for analyzing dose-dependent effects of this compound in animal models?
- Methodological Answer : Apply nonlinear mixed-effects modeling (NONMEM) for pharmacokinetic-pharmacodynamic (PK-PD) data. Account for inter-subject variability using covariates like body weight and organ function. Survival analysis (Kaplan-Meier curves with log-rank tests) is critical for efficacy endpoints. Report 95% confidence intervals and effect sizes to contextualize clinical relevance .
Advanced Research Questions
Q. How can conflicting data on this compound’s mechanism of action be resolved in tubulin-binding assays?
- Methodological Answer : Discrepancies often arise from assay conditions (e.g., buffer pH, GTP concentration). Replicate experiments using standardized protocols (e.g., BRET-based tubulin polymerization assays). Cross-validate with cryo-EM to visualize binding conformations. Perform meta-analyses of published data to identify confounding variables (e.g., cell cycle synchronization methods) .
Q. What experimental strategies address this compound resistance observed in longitudinal in vivo studies?
- Methodological Answer : Profile resistance mechanisms via RNA-seq of treated vs. untreated tumors to identify upregulated efflux pumps (e.g., P-gp) or tubulin isoform shifts. Validate using CRISPR knockouts of candidate genes. Combine this compound with P-gp inhibitors (e.g., verapamil) in co-administration trials. Monitor resistance dynamics with serial biopsies and single-cell sequencing .
Q. How should researchers optimize this compound’s formulation to mitigate off-target toxicity in CNS applications?
- Methodological Answer : Employ blood-brain barrier (BBB) penetration assays (e.g., in vitro PAMPA-BBB models). Test nanoparticle encapsulation (PLGA polymers) or prodrug designs to enhance specificity. Quantify neurotoxicity via glial cell viability assays and in vivo electrophysiology. Use PET imaging with radiolabeled this compound to track biodistribution .
Q. What methodologies reconcile contradictions in this compound’s pharmacokinetic profiles across species?
- Methodological Answer : Perform allometric scaling with species-specific cytochrome P450 activity data. Use physiologically based pharmacokinetic (PBPK) modeling to adjust for metabolic differences. Cross-reference with human hepatocyte assays to predict clearance rates. Publish raw datasets (plasma concentration-time curves) in open-access repositories for independent validation .
Q. Data Analysis and Interpretation
Q. How can researchers systematically review contradictory findings in this compound’s combination therapy trials?
- Methodological Answer : Conduct a PRISMA-guided systematic review with inclusion criteria (e.g., peer-reviewed studies, standardized dosing). Use GRADE criteria to assess evidence quality. Perform network meta-analyses to compare combination regimens (e.g., this compound + gemcitabine vs. This compound + cisplatin). Highlight heterogeneity sources (e.g., patient stratification, endpoint definitions) in sensitivity analyses .
Q. What computational tools are recommended for predicting this compound’s metabolite interactions?
- Methodological Answer : Apply in silico tools like Schrödinger’s ADMET Predictor or SwissADME to identify potential metabolites. Validate with LC-MS/MS fragmentation patterns in hepatic microsome assays. Use molecular docking (AutoDock Vina) to predict metabolite binding to off-target proteins (e.g., hERG channels). Cross-reference with ToxCast databases for toxicity risk assessment .
Q. Ethical and Reproducibility Standards
Q. How can researchers ensure reproducibility in this compound studies amid variability in preclinical models? **
- Methodological Answer : Adopt ARRIVE 2.0 guidelines for animal studies, including detailed reporting of strain, sex, and housing conditions. Share raw data (e.g., tumor volume measurements, flow cytometry files) via platforms like Zenodo. Collaborate via multi-center trials to assess inter-lab variability. Publish negative results to counter publication bias .
特性
CAS番号 |
159776-69-9 |
---|---|
分子式 |
C35H56N6O5 |
分子量 |
640.9 g/mol |
IUPAC名 |
(2S)-N-benzyl-1-[(2S)-1-[(2S)-2-[[(2S)-2-[[(2S)-2-(dimethylamino)-3-methylbutanoyl]amino]-3-methylbutanoyl]-methylamino]-3-methylbutanoyl]pyrrolidine-2-carbonyl]pyrrolidine-2-carboxamide |
InChI |
InChI=1S/C35H56N6O5/c1-22(2)28(37-32(43)29(23(3)4)38(7)8)34(45)39(9)30(24(5)6)35(46)41-20-14-18-27(41)33(44)40-19-13-17-26(40)31(42)36-21-25-15-11-10-12-16-25/h10-12,15-16,22-24,26-30H,13-14,17-21H2,1-9H3,(H,36,42)(H,37,43)/t26-,27-,28-,29-,30-/m0/s1 |
InChIキー |
XSAKVDNHFRWJKS-IIZANFQQSA-N |
SMILES |
CC(C)C(C(=O)N(C)C(C(C)C)C(=O)N1CCCC1C(=O)N2CCCC2C(=O)NCC3=CC=CC=C3)NC(=O)C(C(C)C)N(C)C |
異性体SMILES |
CC(C)[C@@H](C(=O)N(C)[C@@H](C(C)C)C(=O)N1CCC[C@H]1C(=O)N2CCC[C@H]2C(=O)NCC3=CC=CC=C3)NC(=O)[C@H](C(C)C)N(C)C |
正規SMILES |
CC(C)C(C(=O)N(C)C(C(C)C)C(=O)N1CCCC1C(=O)N2CCCC2C(=O)NCC3=CC=CC=C3)NC(=O)C(C(C)C)N(C)C |
配列 |
VVVPP |
同義語 |
cemadotin LU 103793 LU-103793 LU103793 N,N-dimethyl-L-valyl-L-valyl-N-methyl-L-valyl-L-prolyl-L-prolinebenzylamide NSC 669356D NSC D-669356 NSC D669356 NSC-669356D NSC-D669356 |
製品の起源 |
United States |
Retrosynthesis Analysis
AI-Powered Synthesis Planning: Our tool employs the Template_relevance Pistachio, Template_relevance Bkms_metabolic, Template_relevance Pistachio_ringbreaker, Template_relevance Reaxys, Template_relevance Reaxys_biocatalysis model, leveraging a vast database of chemical reactions to predict feasible synthetic routes.
One-Step Synthesis Focus: Specifically designed for one-step synthesis, it provides concise and direct routes for your target compounds, streamlining the synthesis process.
Accurate Predictions: Utilizing the extensive PISTACHIO, BKMS_METABOLIC, PISTACHIO_RINGBREAKER, REAXYS, REAXYS_BIOCATALYSIS database, our tool offers high-accuracy predictions, reflecting the latest in chemical research and data.
Strategy Settings
Precursor scoring | Relevance Heuristic |
---|---|
Min. plausibility | 0.01 |
Model | Template_relevance |
Template Set | Pistachio/Bkms_metabolic/Pistachio_ringbreaker/Reaxys/Reaxys_biocatalysis |
Top-N result to add to graph | 6 |
Feasible Synthetic Routes
試験管内研究製品の免責事項と情報
BenchChemで提示されるすべての記事および製品情報は、情報提供を目的としています。BenchChemで購入可能な製品は、生体外研究のために特別に設計されています。生体外研究は、ラテン語の "in glass" に由来し、生物体の外で行われる実験を指します。これらの製品は医薬品または薬として分類されておらず、FDAから任何の医療状態、病気、または疾患の予防、治療、または治癒のために承認されていません。これらの製品を人間または動物に体内に導入する形態は、法律により厳格に禁止されています。これらのガイドラインに従うことは、研究と実験において法的および倫理的な基準の遵守を確実にするために重要です。