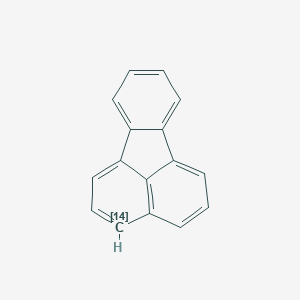
Fluoranthene-3-14C
概要
説明
Fluoranthene-3-14C is a compound with the empirical formula C16H10 . It is a type of polycyclic aromatic hydrocarbon (PAH) that can be viewed as the fusion of naphthalene and benzene unit connected by a five-membered ring . It is colorless and soluble in nonpolar organic solvents .
Synthesis Analysis
Fluoranthene continues to attract significant attention in synthetic organic chemistry and materials science . Recent advances in the synthesis of fluoranthene derivatives have been made . Methods for fluoranthene synthesis are based on strategic bond disconnections .Molecular Structure Analysis
The molecular weight of Fluoranthene-3-14C is 202.25 . The structure of Fluoranthene-3-14C can be represented by the SMILES stringc1ccc-2c (c1)-c3cccc4 [14cH]ccc-2c34
. Chemical Reactions Analysis
Fluoranthene has been used in the synthesis of a variety of fluoranthenes . It has also been used in the analysis of polyaromatic hydrocarbons and polybrominated diphenyl ethers in wastewater .Physical And Chemical Properties Analysis
Fluoranthene-3-14C is a methanol solution with an assay of approximately 95% radiochemical purity . It has a mass shift of M+2 and should be stored at a temperature of 2-8°C . Fluoranthene has a molar mass of 202.256 g·mol−1, a density of 1.252 g/cm3 at 0 °C, a melting point of 110.8 °C, and a boiling point of 375 °C .科学的研究の応用
Biodegradation and Sequestration of Fluoranthene
Direct Link Between Biodegradation and Mobility of Fluoranthene Residues : A study investigated the biodegradation of 14C-fluoranthene in soils and its impact on the distribution of residues among soil compartments. It was found that biodegradation processes controlled the mobility and sequestration of fluoranthene by transforming it into more polar molecules, which could diffuse into intra-aggregate pore water and become bound to the matrix or trapped in microporosity. This highlights the intricate relationship between fluoranthene biodegradation, diffusion, and sequestration in soils (Vessigaud et al., 2007).
Uptake and Distribution in Plants
Root and Foliar Uptake, Translocation, and Distribution of Fluoranthene in Pea Plants : Research demonstrated the uptake of 14C-labeled fluoranthene via roots and leaves of pea plants, showing both acropetal and basipetal transport within the plant. This study provided insights into how fluoranthene distributes within plant systems, which is crucial for understanding the environmental impact and potential phytoremediation strategies (Zezulka et al., 2014).
Metabolism in Plant Cell Cultures and Marine Organisms
Metabolism of Fluoranthene in Plant Cell Cultures and Intact Plants : This study investigated the metabolism of fluoranthene in various plant cell cultures and confirmed that metabolites detected in cell cultures were also formed in intact plants. It highlighted specific metabolites like 1-hydroxyfluoranthene and 8-hydroxy-fluoranthene, providing valuable information on how plants can metabolize and potentially detoxify fluoranthene (Kolb & Harms, 2000).
Biotransformation of Fluoranthene in Marine Polychaete : The study on the polychaete Capitella sp. I revealed how it can accumulate and biotransform fluoranthene, indicating the potential significance of such processes in controlling the fate and effects of PAHs like fluoranthene in contaminated marine sediments (Selck et al., 2003).
Environmental Impact and Remediation Efforts
Transmembrane Transport Theory for Bioremediation of Oil-Polluted Soil : This research introduced the transmembrane transport theory for petroleum hydrocarbons and applied isotopic tracing to understand the transport of PAHs like fluoranthene at a subcellular scale. It also discussed the optimal conditions for the degradation of 14C-fluoranthene, providing insights for bioremediation strategies (Wang et al., 2018).
Potential of the White-Rot Fungus Pleurotus pulmonarius for Degradation of Fluoranthene : This study explored the capability of the white-rot fungus Pleurotus pulmonarius F043 to degrade fluoranthene, emphasizing the importance of the extracellular ligninolytic enzyme system in the biodegradation process. The identification of specific metabolites such as naphthalene-1,8-dicarboxylic acid and phthalic acid during the degradation process shed light on the mechanisms of fluoranthene breakdown by the fungus (Wirasnita & Hadibarata, 2016).
作用機序
Safety and Hazards
将来の方向性
特性
IUPAC Name |
fluoranthene | |
---|---|---|
Source | PubChem | |
URL | https://pubchem.ncbi.nlm.nih.gov | |
Description | Data deposited in or computed by PubChem | |
InChI |
InChI=1S/C16H10/c1-2-8-13-12(7-1)14-9-3-5-11-6-4-10-15(13)16(11)14/h1-10H/i5+2 | |
Source | PubChem | |
URL | https://pubchem.ncbi.nlm.nih.gov | |
Description | Data deposited in or computed by PubChem | |
InChI Key |
GVEPBJHOBDJJJI-RHRFEJLCSA-N | |
Source | PubChem | |
URL | https://pubchem.ncbi.nlm.nih.gov | |
Description | Data deposited in or computed by PubChem | |
Canonical SMILES |
C1=CC=C2C(=C1)C3=CC=CC4=C3C2=CC=C4 | |
Source | PubChem | |
URL | https://pubchem.ncbi.nlm.nih.gov | |
Description | Data deposited in or computed by PubChem | |
Isomeric SMILES |
C1=CC=C2C(=C1)C3=CC=CC4=C3C2=CC=[14CH]4 | |
Source | PubChem | |
URL | https://pubchem.ncbi.nlm.nih.gov | |
Description | Data deposited in or computed by PubChem | |
Molecular Formula |
C16H10 | |
Source | PubChem | |
URL | https://pubchem.ncbi.nlm.nih.gov | |
Description | Data deposited in or computed by PubChem | |
DSSTOX Substance ID |
DTXSID10585156 | |
Record name | (3-~14~C)Fluoranthene | |
Source | EPA DSSTox | |
URL | https://comptox.epa.gov/dashboard/DTXSID10585156 | |
Description | DSSTox provides a high quality public chemistry resource for supporting improved predictive toxicology. | |
Molecular Weight |
204.24 g/mol | |
Source | PubChem | |
URL | https://pubchem.ncbi.nlm.nih.gov | |
Description | Data deposited in or computed by PubChem | |
Product Name |
Fluoranthene-3-14C | |
CAS RN |
134459-04-4 | |
Record name | (3-~14~C)Fluoranthene | |
Source | EPA DSSTox | |
URL | https://comptox.epa.gov/dashboard/DTXSID10585156 | |
Description | DSSTox provides a high quality public chemistry resource for supporting improved predictive toxicology. | |
Retrosynthesis Analysis
AI-Powered Synthesis Planning: Our tool employs the Template_relevance Pistachio, Template_relevance Bkms_metabolic, Template_relevance Pistachio_ringbreaker, Template_relevance Reaxys, Template_relevance Reaxys_biocatalysis model, leveraging a vast database of chemical reactions to predict feasible synthetic routes.
One-Step Synthesis Focus: Specifically designed for one-step synthesis, it provides concise and direct routes for your target compounds, streamlining the synthesis process.
Accurate Predictions: Utilizing the extensive PISTACHIO, BKMS_METABOLIC, PISTACHIO_RINGBREAKER, REAXYS, REAXYS_BIOCATALYSIS database, our tool offers high-accuracy predictions, reflecting the latest in chemical research and data.
Strategy Settings
Precursor scoring | Relevance Heuristic |
---|---|
Min. plausibility | 0.01 |
Model | Template_relevance |
Template Set | Pistachio/Bkms_metabolic/Pistachio_ringbreaker/Reaxys/Reaxys_biocatalysis |
Top-N result to add to graph | 6 |
Feasible Synthetic Routes
試験管内研究製品の免責事項と情報
BenchChemで提示されるすべての記事および製品情報は、情報提供を目的としています。BenchChemで購入可能な製品は、生体外研究のために特別に設計されています。生体外研究は、ラテン語の "in glass" に由来し、生物体の外で行われる実験を指します。これらの製品は医薬品または薬として分類されておらず、FDAから任何の医療状態、病気、または疾患の予防、治療、または治癒のために承認されていません。これらの製品を人間または動物に体内に導入する形態は、法律により厳格に禁止されています。これらのガイドラインに従うことは、研究と実験において法的および倫理的な基準の遵守を確実にするために重要です。