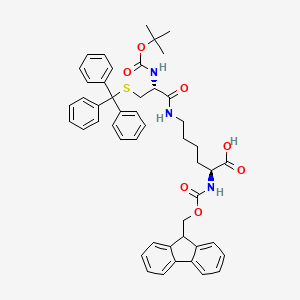
Fmoc-lys(boc-cys(trt))-OH
概要
説明
Fmoc-Lys(Boc-Cys(Trt))-OH is a protected amino acid derivative widely used in solid-phase peptide synthesis (SPPS). It features three orthogonal protecting groups:
- Fmoc (9-fluorenylmethyloxycarbonyl) on the α-amino group of lysine.
- Boc (tert-butyloxycarbonyl) on the ε-amino group of lysine.
- Trt (trityl) on the thiol group of cysteine, which is attached to the lysine side chain.
This compound enables selective deprotection during peptide assembly, particularly for constructing branched or cyclic peptides with cysteine-mediated disulfide bonds . Its synthesis involves coupling Boc-Cys(Trt)-OSu to Fmoc-Lys-OH in methanol using DIPEA as a base, followed by purification via flash chromatography (yield: 80%) .
準備方法
Synthetic Routes and Reaction Conditions
The synthesis of Fmoc-lys(boc-cys(trt))-OH typically involves solid-phase peptide synthesis (SPPS) using Fmoc/tBu strategy. The process begins with the attachment of the first amino acid to a solid resin. The Fmoc group is then removed using piperidine, allowing the next amino acid to be coupled. This cycle of deprotection and coupling continues until the desired peptide sequence is obtained. The Boc and Trt groups are used to protect the side chains of lysine and cysteine during the synthesis .
Industrial Production Methods
Industrial production of this compound follows similar principles as laboratory synthesis but on a larger scale. Automated peptide synthesizers are often used to increase efficiency and reproducibility. The use of high-performance liquid chromatography (HPLC) is common for purifying the final product .
化学反応の分析
Types of Reactions
Fmoc-lys(boc-cys(trt))-OH undergoes several types of chemical reactions, including:
Deprotection Reactions: Removal of Fmoc, Boc, and Trt groups using specific reagents.
Coupling Reactions: Formation of peptide bonds with other amino acids.
Oxidation and Reduction: Modifications of the cysteine thiol group.
Common Reagents and Conditions
Fmoc Deprotection: Piperidine in dimethylformamide (DMF).
Boc Deprotection: Trifluoroacetic acid (TFA).
Trt Deprotection: TFA with scavengers like triisopropylsilane (TIPS) and water.
Major Products Formed
The major products formed from these reactions are peptides with specific sequences, where the protecting groups have been selectively removed to allow for further modifications or applications .
科学的研究の応用
Peptide Synthesis
Solid-Phase Peptide Synthesis (SPPS) : Fmoc-Lys(Boc-Cys(Trt))-OH is primarily used in SPPS due to its protective groups that allow for selective reactions. The Fmoc group can be easily removed under mild basic conditions, facilitating the stepwise addition of amino acids. The use of this compound enhances the efficiency of synthesizing complex peptides by minimizing side reactions and ensuring high yields .
Case Study : A study demonstrated the successful incorporation of this compound in synthesizing cyclic peptides through tandem N-to-S acyl migration and native chemical ligation. This method showcased the compound's ability to streamline the synthesis process while maintaining peptide integrity .
Drug Development
Peptide-Based Therapeutics : In pharmaceutical research, this compound is pivotal for developing peptide-based drugs. Its unique structure allows researchers to design molecules that can target specific biological pathways effectively. This capability is crucial for creating innovative therapies for various diseases, including cancer and autoimmune disorders .
Example Application : Research has indicated that derivatives of peptides synthesized using this compound exhibit enhanced bioactivity and stability, making them promising candidates for drug development .
Bioconjugation
Enhancing Delivery Mechanisms : The compound plays a significant role in bioconjugation processes, where it facilitates the attachment of peptides to biomolecules such as antibodies or nanoparticles. This conjugation improves the delivery and efficacy of therapeutic agents by enhancing their stability and targeting capabilities .
Research Insight : A recent review highlighted various applications of Fmoc-protected cysteine derivatives in bioconjugation, emphasizing their role in improving the pharmacokinetic properties of therapeutic peptides .
Diagnostics
Development of Diagnostic Tools : this compound is instrumental in creating diagnostic assays that rely on specific peptide interactions. By incorporating this compound into assay designs, researchers can enhance the sensitivity and specificity of tests used in clinical settings .
Case Study Example : The integration of this compound in diagnostic assays has been shown to improve detection limits and reduce background noise in immunoassays, making it a valuable tool for early disease detection .
Research in Protein Engineering
Tailoring Protein Functionality : In protein engineering studies, this compound allows researchers to introduce specific amino acids into proteins, enabling modifications that enhance functionality for various applications in biotechnology .
Notable Research Findings : Investigations have demonstrated that proteins modified with this compound exhibit improved characteristics such as increased stability and activity, which are critical for industrial applications .
Data Tables
作用機序
The mechanism of action of Fmoc-lys(boc-cys(trt))-OH involves the selective protection and deprotection of amino acid side chains during peptide synthesis. The Fmoc group protects the amino group of lysine, while the Boc and Trt groups protect the side chains of lysine and cysteine, respectively. These protecting groups prevent unwanted side reactions, allowing for the precise assembly of peptide sequences.
類似化合物との比較
Comparison with Structurally Similar Compounds
Fmoc-Lys(Boc)-OH
- Structural Difference : Lacks the cysteine side chain and Trt protection.
- Synthesis: Direct Boc protection of lysine’s ε-amino group using Boc₂O .
- Applications : Used in linear peptide synthesis and dendrimer construction. In contrast, Fmoc-Lys(Boc-Cys(Trt))-OH is tailored for introducing disulfide bonds or thiol-reactive sites .
- Purity : Commercial batches achieve >98% purity (HPLC), similar to this compound .
Fmoc-Cys(Trt)-OH
- Structural Difference : A cysteine derivative without lysine or Boc protection.
- Applications : Used for fluorescence-based detection of antibiotics (e.g., ofloxacin) due to its Trt-mediated energy transfer properties . Unlike this compound, it lacks versatility in branching but excels in sensor applications.
- Purity : ≥98.5% by HPLC, with residual impurities (e.g., Trt-Cl, Trt-OH) tightly controlled .
Fmoc-Lys(Trt)-OH
- Structural Difference: Replaces Boc-Cys(Trt) with Trt on lysine’s ε-amino group.
- Applications: Ideal for synthesizing peptides requiring acid-labile Trt deprotection. However, it cannot form disulfide bonds, limiting its utility in cyclization compared to this compound .
- Solubility: 4.69 mg/mL in water vs. 51 mg/mL in ethanol, highlighting solvent compatibility differences with Boc-Cys(Trt)-modified analogs .
Cyclic Lysine Dipeptides (e.g., Fmoc-Lys(Boc)-Cyclic Dipeptide)
- Structural Difference : Cyclic backbone with dual Fmoc/Boc protection.
- Gelation Properties : Forms thermoreversible gels in alcohols and chlorinated solvents (MGC: 1–5% w/v). Hydrogen bonds dominate in chlorinated solvents, while π-π stacking drives assembly in alcohols .
- Comparison: this compound lacks inherent gelation but offers thiol reactivity for post-synthetic modifications (e.g., nanoparticle conjugation) .
Functional and Analytical Comparison
Physicochemical Properties
Research Implications and Limitations
- Advantages of this compound : Combines thiol reactivity with orthogonal protection, enabling complex peptide architectures.
- Limitations: Higher molecular weight (vs. Fmoc-Lys(Boc)-OH) may reduce solubility in non-polar solvents.
- Future Directions : Optimize deprotection protocols to minimize side reactions and expand applications in bioconjugation .
生物活性
Fmoc-lys(boc-cys(trt))-OH is a synthetic amino acid derivative that combines Fmoc (9-fluorenylmethoxycarbonyl) protection with lysine and cysteine functionalities. This compound is of significant interest in peptide synthesis and biological research due to its unique properties that facilitate the formation of complex peptides, particularly those involving disulfide bonds. Below is a detailed examination of its biological activity, including relevant data tables and case studies.
Chemical Structure and Properties
- Molecular Formula : C₄₈H₅₁N₃O₇S
- Molecular Weight : 814 g/mol
- Purity : ≥ 99.5% (HPLC)
The compound features a lysine residue with a Boc (tert-butyloxycarbonyl) protecting group on its amino side chain, and a cysteine residue protected by a Trt (trityl) group. This configuration allows for selective deprotection and subsequent reactions, making it ideal for solid-phase peptide synthesis (SPPS) .
1. Peptide Synthesis Applications
This compound is primarily used in the synthesis of peptides that require the incorporation of cysteine for disulfide bond formation. The Trt group can be selectively removed under mild acidic conditions, allowing for the formation of cyclic peptides or peptides with multiple disulfide linkages .
Table 1: Comparison of Protecting Groups in SPPS
Protecting Group | Stability | Deprotection Conditions | Application |
---|---|---|---|
Trt | Moderate | 95% TFA + TIS | Disulfide formation |
Boc | High | 50% TFA | General peptide synthesis |
Acm | Low | Mild Acid | Sensitive peptides |
2. Case Studies
Case Study 1: Synthesis of Cyclic Peptides
A study demonstrated the use of this compound in the synthesis of cyclic peptides through tandem N-to-S acyl migration. This method showcased the efficiency of using this compound to create cyclic structures without requiring harsh conditions, thus preserving the integrity of sensitive residues .
Case Study 2: Selective Amide Bond Cleavage
In another research project, this compound was utilized to explore selective bi-directional amide bond cleavage in peptides. This study provided insights into controlled peptide modifications, which are crucial for developing therapeutic peptides .
The biological activity of this compound can be attributed to its ability to facilitate specific interactions within peptide chains. The presence of cysteine allows for the formation of disulfide bonds, which are critical for maintaining the structural integrity and biological function of many proteins.
3. Racemization Prevention
One significant challenge in peptide synthesis involving cysteine derivatives is racemization. Research has shown that using this compound with specific coupling reagents can minimize racemization rates during synthesis, ensuring higher yields of the desired enantiomer .
Q & A
Basic Research Questions
Q. How should Fmoc-Lys(Boc-Cys(Trt))-OH be incorporated into solid-phase peptide synthesis (SPPS) protocols?
- Methodological Answer : this compound requires sequential deprotection strategies. The Fmoc group on the α-amino is removed using 20% piperidine in DMF, while the Boc group on Lys and Trt on Cys remain intact. Post-synthesis, the Boc group is cleaved with 95% trifluoroacetic acid (TFA), and the Trt group on Cys is removed under milder acidic conditions (e.g., 1% TFA in dichloromethane). Ensure orthogonal protection to avoid premature deprotection .
Q. What analytical methods are critical for verifying the purity and stereochemical integrity of this compound?
- Methodological Answer : Use reversed-phase HPLC (≥99% purity) to assess chemical purity and chiral chromatography (≥99.8% enantiomeric purity) to confirm stereochemical integrity. Mass spectrometry (MS) validates molecular weight. For stability, monitor thermal decomposition via differential scanning calorimetry (DSC) and ensure storage at -20°C in anhydrous conditions .
Q. How should researchers handle and store this compound to prevent degradation?
- Methodological Answer : Store lyophilized powder under inert gas (argon/nitrogen) at -20°C, shielded from light and moisture. Reconstitute in dry DMF or DCM immediately before use. Avoid prolonged exposure to basic conditions (e.g., piperidine) to prevent Trt-group migration .
Advanced Research Questions
Q. How can discrepancies in nanoparticle size distributions during Fmoc-Cys(Trt)-OH-based drug delivery system synthesis be resolved?
- Methodological Answer : Particle size variability (e.g., 243–750 nm) arises from synthesis methods (manual vs. microfluidic) and concentration effects . Optimize flow rates in microfluidic devices to enhance mixing homogeneity. For manual synthesis, use sonication to reduce agglomeration. Validate reproducibility via dynamic light scattering (DLS) across three independent batches .
Q. What strategies prevent side reactions during orthogonal deprotection of Boc and Trt groups in complex peptide sequences?
- Methodological Answer : Use scavengers (e.g., triisopropylsilane) during Boc deprotection to minimize carbocation-mediated side reactions. For Trt removal, employ low-temperature (0–4°C) acidic conditions (1% TFA) to reduce acidolysis of adjacent groups. Monitor reaction progress via LC-MS to detect incomplete deprotection or Trt migration .
Q. How can this compound be applied in pH-responsive drug delivery systems?
- Methodological Answer : The Trt group on Cys enables pH-sensitive disulfide bond formation in core-shell nanoparticles. At tumor microenvironment pH (6.5–7.0), Trt deprotection triggers drug release. Design microfluidic reactors to control particle size (542 nm optimal for cellular uptake) and polydispersity (PDI <0.1) .
Q. Data Contradiction Analysis
Q. Why do manual and microfluidic synthesis methods yield opposing trends in nanoparticle size for Fmoc-Cys(Trt)-OH?
- Methodological Answer : Manual agitation at 0.1 mg/mL produces larger particles (750 nm) due to uneven mixing, while microfluidic flow (Re > 200) reduces aggregation (542 nm). At higher concentrations (0.5 mg/mL), manual methods favor smaller particles via kinetic trapping, whereas microfluidic systems stabilize intermediate sizes. Reconcile data by standardizing shear stress and residence time .
Q. Experimental Design Considerations
Q. What steps ensure reproducibility in peptide conjugates containing this compound?
- Methodological Answer : Pre-activate the carboxyl group with HBTU/HOBt in DMF for 5 min before coupling. Use a 3-fold molar excess of this compound relative to resin-bound peptide. Confirm coupling efficiency via Kaiser test or FT-IR for unreacted amines. For long sequences, incorporate pseudoproline dipeptides to reduce aggregation .
Q. Tables for Key Data
Table 1 : Nanoparticle Size and PDI Under Varying Synthesis Conditions
Concentration (mg/mL) | Method | Avg. Size (nm) | PDI |
---|---|---|---|
0.1 | Manual | 750 | 0.09 ± 0.008 |
0.1 | Microfluidic | 542 | 0.26 ± 0.07 |
0.5 | Manual | 510 | 0.15 ± 0.02 |
0.5 | Microfluidic | 550 | 0.12 ± 0.03 |
Table 2 : Deprotection Conditions for Key Protecting Groups
Protecting Group | Cleavage Reagent | Time (min) | Temperature |
---|---|---|---|
Fmoc | 20% piperidine/DMF | 10–20 | RT |
Boc | 95% TFA + 2.5% H2O | 30 | RT |
Trt (Cys) | 1% TFA/DCM + 2% triisopropylsilane | 10 | 0–4°C |
特性
IUPAC Name |
(2S)-2-(9H-fluoren-9-ylmethoxycarbonylamino)-6-[[(2R)-2-[(2-methylpropan-2-yl)oxycarbonylamino]-3-tritylsulfanylpropanoyl]amino]hexanoic acid | |
---|---|---|
Source | PubChem | |
URL | https://pubchem.ncbi.nlm.nih.gov | |
Description | Data deposited in or computed by PubChem | |
InChI |
InChI=1S/C48H51N3O7S/c1-47(2,3)58-46(56)51-42(32-59-48(33-19-7-4-8-20-33,34-21-9-5-10-22-34)35-23-11-6-12-24-35)43(52)49-30-18-17-29-41(44(53)54)50-45(55)57-31-40-38-27-15-13-25-36(38)37-26-14-16-28-39(37)40/h4-16,19-28,40-42H,17-18,29-32H2,1-3H3,(H,49,52)(H,50,55)(H,51,56)(H,53,54)/t41-,42-/m0/s1 | |
Source | PubChem | |
URL | https://pubchem.ncbi.nlm.nih.gov | |
Description | Data deposited in or computed by PubChem | |
InChI Key |
DKIRZVVHIJMLBK-COCZKOEFSA-N | |
Source | PubChem | |
URL | https://pubchem.ncbi.nlm.nih.gov | |
Description | Data deposited in or computed by PubChem | |
Canonical SMILES |
CC(C)(C)OC(=O)NC(CSC(C1=CC=CC=C1)(C2=CC=CC=C2)C3=CC=CC=C3)C(=O)NCCCCC(C(=O)O)NC(=O)OCC4C5=CC=CC=C5C6=CC=CC=C46 | |
Source | PubChem | |
URL | https://pubchem.ncbi.nlm.nih.gov | |
Description | Data deposited in or computed by PubChem | |
Isomeric SMILES |
CC(C)(C)OC(=O)N[C@@H](CSC(C1=CC=CC=C1)(C2=CC=CC=C2)C3=CC=CC=C3)C(=O)NCCCC[C@@H](C(=O)O)NC(=O)OCC4C5=CC=CC=C5C6=CC=CC=C46 | |
Source | PubChem | |
URL | https://pubchem.ncbi.nlm.nih.gov | |
Description | Data deposited in or computed by PubChem | |
Molecular Formula |
C48H51N3O7S | |
Source | PubChem | |
URL | https://pubchem.ncbi.nlm.nih.gov | |
Description | Data deposited in or computed by PubChem | |
Molecular Weight |
814.0 g/mol | |
Source | PubChem | |
URL | https://pubchem.ncbi.nlm.nih.gov | |
Description | Data deposited in or computed by PubChem | |
Retrosynthesis Analysis
AI-Powered Synthesis Planning: Our tool employs the Template_relevance Pistachio, Template_relevance Bkms_metabolic, Template_relevance Pistachio_ringbreaker, Template_relevance Reaxys, Template_relevance Reaxys_biocatalysis model, leveraging a vast database of chemical reactions to predict feasible synthetic routes.
One-Step Synthesis Focus: Specifically designed for one-step synthesis, it provides concise and direct routes for your target compounds, streamlining the synthesis process.
Accurate Predictions: Utilizing the extensive PISTACHIO, BKMS_METABOLIC, PISTACHIO_RINGBREAKER, REAXYS, REAXYS_BIOCATALYSIS database, our tool offers high-accuracy predictions, reflecting the latest in chemical research and data.
Strategy Settings
Precursor scoring | Relevance Heuristic |
---|---|
Min. plausibility | 0.01 |
Model | Template_relevance |
Template Set | Pistachio/Bkms_metabolic/Pistachio_ringbreaker/Reaxys/Reaxys_biocatalysis |
Top-N result to add to graph | 6 |
Feasible Synthetic Routes
試験管内研究製品の免責事項と情報
BenchChemで提示されるすべての記事および製品情報は、情報提供を目的としています。BenchChemで購入可能な製品は、生体外研究のために特別に設計されています。生体外研究は、ラテン語の "in glass" に由来し、生物体の外で行われる実験を指します。これらの製品は医薬品または薬として分類されておらず、FDAから任何の医療状態、病気、または疾患の予防、治療、または治癒のために承認されていません。これらの製品を人間または動物に体内に導入する形態は、法律により厳格に禁止されています。これらのガイドラインに従うことは、研究と実験において法的および倫理的な基準の遵守を確実にするために重要です。