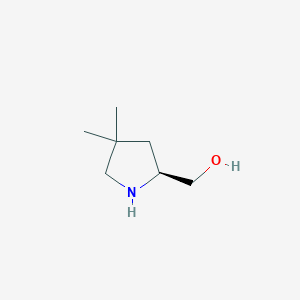
(S)-(4,4-Dimethylpyrrolidin-2-yl)methanol
概要
説明
(S)-(4,4-Dimethylpyrrolidin-2-yl)methanol is a chiral alcohol compound with a pyrrolidine ring substituted with a hydroxymethyl group at the 2-position and two methyl groups at the 4-position
準備方法
Synthetic Routes and Reaction Conditions
The synthesis of (S)-(4,4-Dimethylpyrrolidin-2-yl)methanol typically involves the reduction of the corresponding ketone or aldehyde precursor. One common method is the reduction of (S)-4,4-dimethylpyrrolidin-2-one using a reducing agent such as sodium borohydride (NaBH4) or lithium aluminum hydride (LiAlH4) under controlled conditions . The reaction is usually carried out in an inert solvent like tetrahydrofuran (THF) or diethyl ether at low temperatures to ensure high yield and selectivity.
Industrial Production Methods
In an industrial setting, the production of this compound may involve catalytic hydrogenation of the corresponding ketone using a metal catalyst such as palladium on carbon (Pd/C) under high pressure and temperature . This method allows for efficient large-scale production with high enantiomeric purity.
化学反応の分析
Types of Reactions
(S)-(4,4-Dimethylpyrrolidin-2-yl)methanol can undergo various chemical reactions, including:
Oxidation: The hydroxyl group can be oxidized to form the corresponding ketone or aldehyde using oxidizing agents like pyridinium chlorochromate (PCC) or Jones reagent.
Reduction: The compound can be further reduced to form the corresponding alkane using strong reducing agents.
Substitution: The hydroxyl group can be substituted with other functional groups through nucleophilic substitution reactions.
Common Reagents and Conditions
Oxidation: PCC, Jones reagent, or Dess-Martin periodinane in solvents like dichloromethane (DCM).
Reduction: LiAlH4 or NaBH4 in THF or diethyl ether.
Substitution: Tosyl chloride (TsCl) in pyridine for conversion to tosylate, followed by nucleophiles like sodium azide (NaN3) or potassium cyanide (KCN).
Major Products
Oxidation: (S)-4,4-Dimethylpyrrolidin-2-one.
Reduction: (S)-4,4-Dimethylpyrrolidine.
Substitution: Various substituted derivatives depending on the nucleophile used.
科学的研究の応用
(S)-(4,4-Dimethylpyrrolidin-2-yl)methanol has several applications in scientific research:
Chemistry: Used as a chiral building block in the synthesis of complex organic molecules.
Biology: Investigated for its potential as a chiral ligand in asymmetric synthesis and catalysis.
Medicine: Explored for its potential use in the development of pharmaceuticals, particularly as intermediates in the synthesis of active pharmaceutical ingredients (APIs).
作用機序
The mechanism of action of (S)-(4,4-Dimethylpyrrolidin-2-yl)methanol depends on its specific application. In asymmetric synthesis, it acts as a chiral auxiliary or ligand, facilitating the formation of enantiomerically pure products by coordinating with metal catalysts and directing the stereochemistry of the reaction . The molecular targets and pathways involved vary based on the specific reactions and catalysts used.
類似化合物との比較
Similar Compounds
®-(4,4-Dimethylpyrrolidin-2-yl)methanol: The enantiomer of the compound with similar chemical properties but different stereochemistry.
4,4-Dimethylpyrrolidin-2-one: The ketone precursor used in the synthesis of (S)-(4,4-Dimethylpyrrolidin-2-yl)methanol.
4,4-Dimethylpyrrolidine: The fully reduced form of the compound.
Uniqueness
This compound is unique due to its chiral nature, which makes it valuable in asymmetric synthesis and the production of enantiomerically pure compounds. Its specific stereochemistry allows for selective interactions with chiral catalysts and reagents, making it a versatile tool in organic synthesis .
特性
IUPAC Name |
[(2S)-4,4-dimethylpyrrolidin-2-yl]methanol | |
---|---|---|
Source | PubChem | |
URL | https://pubchem.ncbi.nlm.nih.gov | |
Description | Data deposited in or computed by PubChem | |
InChI |
InChI=1S/C7H15NO/c1-7(2)3-6(4-9)8-5-7/h6,8-9H,3-5H2,1-2H3/t6-/m0/s1 | |
Source | PubChem | |
URL | https://pubchem.ncbi.nlm.nih.gov | |
Description | Data deposited in or computed by PubChem | |
InChI Key |
DJIQDHXAMYMRRC-LURJTMIESA-N | |
Source | PubChem | |
URL | https://pubchem.ncbi.nlm.nih.gov | |
Description | Data deposited in or computed by PubChem | |
Canonical SMILES |
CC1(CC(NC1)CO)C | |
Source | PubChem | |
URL | https://pubchem.ncbi.nlm.nih.gov | |
Description | Data deposited in or computed by PubChem | |
Isomeric SMILES |
CC1(C[C@H](NC1)CO)C | |
Source | PubChem | |
URL | https://pubchem.ncbi.nlm.nih.gov | |
Description | Data deposited in or computed by PubChem | |
Molecular Formula |
C7H15NO | |
Source | PubChem | |
URL | https://pubchem.ncbi.nlm.nih.gov | |
Description | Data deposited in or computed by PubChem | |
Molecular Weight |
129.20 g/mol | |
Source | PubChem | |
URL | https://pubchem.ncbi.nlm.nih.gov | |
Description | Data deposited in or computed by PubChem | |
Retrosynthesis Analysis
AI-Powered Synthesis Planning: Our tool employs the Template_relevance Pistachio, Template_relevance Bkms_metabolic, Template_relevance Pistachio_ringbreaker, Template_relevance Reaxys, Template_relevance Reaxys_biocatalysis model, leveraging a vast database of chemical reactions to predict feasible synthetic routes.
One-Step Synthesis Focus: Specifically designed for one-step synthesis, it provides concise and direct routes for your target compounds, streamlining the synthesis process.
Accurate Predictions: Utilizing the extensive PISTACHIO, BKMS_METABOLIC, PISTACHIO_RINGBREAKER, REAXYS, REAXYS_BIOCATALYSIS database, our tool offers high-accuracy predictions, reflecting the latest in chemical research and data.
Strategy Settings
Precursor scoring | Relevance Heuristic |
---|---|
Min. plausibility | 0.01 |
Model | Template_relevance |
Template Set | Pistachio/Bkms_metabolic/Pistachio_ringbreaker/Reaxys/Reaxys_biocatalysis |
Top-N result to add to graph | 6 |
Feasible Synthetic Routes
試験管内研究製品の免責事項と情報
BenchChemで提示されるすべての記事および製品情報は、情報提供を目的としています。BenchChemで購入可能な製品は、生体外研究のために特別に設計されています。生体外研究は、ラテン語の "in glass" に由来し、生物体の外で行われる実験を指します。これらの製品は医薬品または薬として分類されておらず、FDAから任何の医療状態、病気、または疾患の予防、治療、または治癒のために承認されていません。これらの製品を人間または動物に体内に導入する形態は、法律により厳格に禁止されています。これらのガイドラインに従うことは、研究と実験において法的および倫理的な基準の遵守を確実にするために重要です。