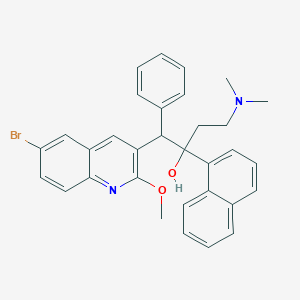
1-(6-Bromo-2-methoxy-3-quinolyl)-4-(dimethylamino)-2-(1-naphthyl)-1-phenyl-butan-2-ol
説明
The compound "1-(6-Bromo-2-methoxy-3-quinolyl)-4-(dimethylamino)-2-(1-naphthyl)-1-phenyl-butan-2-ol" is a complex organic molecule that appears to be related to various research areas, including photophysics, anti-inflammatory activity, and synthetic organic chemistry. The compound's structure suggests it contains a quinoline moiety, which is a common scaffold in medicinal chemistry due to its pharmacological properties .
Synthesis Analysis
The synthesis of related compounds often involves halogenated intermediates, as seen in the preparation of 2-bromo-6-methoxynaphthalene, an important intermediate for anti-inflammatory agents . The synthesis of similar compounds has been achieved through various methods, including methylation reactions and bromination techniques. For instance, asymmetric bromination has been used to synthesize chiral derivatives of naphthalene compounds . Although the exact synthesis of the compound is not detailed in the provided papers, these methods could potentially be adapted for its synthesis.
Molecular Structure Analysis
The molecular structure of related compounds has been studied using techniques such as X-ray diffraction, which provides information on the crystal system, space group, and dihedral angles between different moieties within the molecule . The presence of weak hydrogen bonds, such as C–H···O, C–H···N, and C–H···Br, can contribute to the stabilization of the three-dimensional structure of these molecules .
Chemical Reactions Analysis
The compound's reactivity can be inferred from related studies. For example, amino derivatives of pyrazoloquinoline exhibit solvatochromism, acidochromism, and can participate in electron transfer processes . These properties suggest that the compound may also exhibit interesting photophysical properties and could potentially be used in molecular logic switches or as a fluorescent probe, depending on its specific substituents and reaction conditions.
Physical and Chemical Properties Analysis
The physical and chemical properties of such compounds are influenced by their molecular structure. The presence of a bromo and methoxy group on the quinoline and naphthyl rings, respectively, can affect the molecule's lipophilicity, which is a significant factor in anti-inflammatory activity . The introduction of specific functional groups can either enhance or diminish the biological activity of these compounds, as seen in the structure-activity relationship studies of anti-inflammatory analogues .
科学的研究の応用
Antituberculosis Activity
New 4-dimethylamino-2-(naphthalen-1-yl)-1-phenyl-1-(quinolin-3-yl)butan-2-ols, including 1-(6-Bromo-2-methoxy-3-quinolyl)-4-(dimethylamino)-2-(1-naphthyl)-1-phenyl-butan-2-ol, have been synthesized and found to exhibit significant antituberculosis activity. One of these compounds is in the final stage of clinical trials and is being prepared for use in clinical practice (Omel’kov, Fedorov, & Stepanov, 2019).
Structural and Molecular Analysis
The compound's structure is characterized by unique molecular arrangements and interactions, such as Br-Br interactions and π-stacking, which play a role in its structural cohesion. These features may influence its biological activity and could be a focal point for further research (Petit, Coquerel, Meyer, & Guillemont, 2007).
Development of Anti-TB Drug TMS-207
The discovery of highly active compounds against Mycobacterium Tuberculosis and its drug-resistant strains led to the creation of the innovative anti-TB drug TMS-207 in 2013. This development was based on derivatives of 1-(6-Bromo-2-methoxy-3-quinolyl)-4-(dimethylamino)-2-(naphth-1-yl)-1-phenyl-butane-2-ol, highlighting its crucial role in addressing tuberculosis (Omelkov, Ruchko, & Fedorov, 2020).
Safety And Hazards
特性
IUPAC Name |
1-(6-bromo-2-methoxyquinolin-3-yl)-4-(dimethylamino)-2-naphthalen-1-yl-1-phenylbutan-2-ol | |
---|---|---|
Source | PubChem | |
URL | https://pubchem.ncbi.nlm.nih.gov | |
Description | Data deposited in or computed by PubChem | |
InChI |
InChI=1S/C32H31BrN2O2/c1-35(2)19-18-32(36,28-15-9-13-22-10-7-8-14-26(22)28)30(23-11-5-4-6-12-23)27-21-24-20-25(33)16-17-29(24)34-31(27)37-3/h4-17,20-21,30,36H,18-19H2,1-3H3 | |
Source | PubChem | |
URL | https://pubchem.ncbi.nlm.nih.gov | |
Description | Data deposited in or computed by PubChem | |
InChI Key |
QUIJNHUBAXPXFS-UHFFFAOYSA-N | |
Source | PubChem | |
URL | https://pubchem.ncbi.nlm.nih.gov | |
Description | Data deposited in or computed by PubChem | |
Canonical SMILES |
CN(C)CCC(C1=CC=CC2=CC=CC=C21)(C(C3=CC=CC=C3)C4=C(N=C5C=CC(=CC5=C4)Br)OC)O | |
Source | PubChem | |
URL | https://pubchem.ncbi.nlm.nih.gov | |
Description | Data deposited in or computed by PubChem | |
Molecular Formula |
C32H31BrN2O2 | |
Source | PubChem | |
URL | https://pubchem.ncbi.nlm.nih.gov | |
Description | Data deposited in or computed by PubChem | |
Molecular Weight |
555.5 g/mol | |
Source | PubChem | |
URL | https://pubchem.ncbi.nlm.nih.gov | |
Description | Data deposited in or computed by PubChem | |
Product Name |
1-(6-Bromo-2-methoxy-3-quinolyl)-4-(dimethylamino)-2-(1-naphthyl)-1-phenyl-butan-2-ol |
Retrosynthesis Analysis
AI-Powered Synthesis Planning: Our tool employs the Template_relevance Pistachio, Template_relevance Bkms_metabolic, Template_relevance Pistachio_ringbreaker, Template_relevance Reaxys, Template_relevance Reaxys_biocatalysis model, leveraging a vast database of chemical reactions to predict feasible synthetic routes.
One-Step Synthesis Focus: Specifically designed for one-step synthesis, it provides concise and direct routes for your target compounds, streamlining the synthesis process.
Accurate Predictions: Utilizing the extensive PISTACHIO, BKMS_METABOLIC, PISTACHIO_RINGBREAKER, REAXYS, REAXYS_BIOCATALYSIS database, our tool offers high-accuracy predictions, reflecting the latest in chemical research and data.
Strategy Settings
Precursor scoring | Relevance Heuristic |
---|---|
Min. plausibility | 0.01 |
Model | Template_relevance |
Template Set | Pistachio/Bkms_metabolic/Pistachio_ringbreaker/Reaxys/Reaxys_biocatalysis |
Top-N result to add to graph | 6 |
Feasible Synthetic Routes
試験管内研究製品の免責事項と情報
BenchChemで提示されるすべての記事および製品情報は、情報提供を目的としています。BenchChemで購入可能な製品は、生体外研究のために特別に設計されています。生体外研究は、ラテン語の "in glass" に由来し、生物体の外で行われる実験を指します。これらの製品は医薬品または薬として分類されておらず、FDAから任何の医療状態、病気、または疾患の予防、治療、または治癒のために承認されていません。これらの製品を人間または動物に体内に導入する形態は、法律により厳格に禁止されています。これらのガイドラインに従うことは、研究と実験において法的および倫理的な基準の遵守を確実にするために重要です。