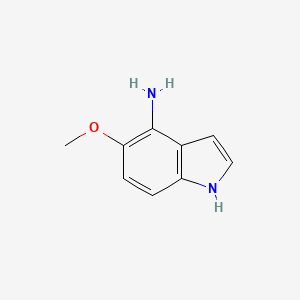
5-methoxy-1H-indol-4-amine
概要
説明
5-Methoxy-1H-indol-4-amine is a chemical compound belonging to the indole family. Indoles are heterocyclic compounds that are widely studied due to their presence in many natural products and pharmaceuticals. The structure of this compound consists of an indole core with a methoxy group at the 5-position and an amine group at the 4-position.
科学的研究の応用
5-Methoxy-1H-indol-4-amine has a wide range of applications in scientific research:
Chemistry: It serves as a building block for the synthesis of more complex indole derivatives.
Medicine: Research explores its potential as a therapeutic agent for various diseases.
Industry: It is used in the development of dyes, pigments, and other industrial chemicals.
作用機序
Target of Action
5-Methoxy-1H-indol-4-amine, like many indole derivatives, has been found to bind with high affinity to multiple receptors . These receptors are often the primary targets of the compound, and their role is crucial in the compound’s biological activity.
Mode of Action
It is known that indole derivatives interact with their targets, causing changes that lead to various biological activities . These activities can include antiviral, anti-inflammatory, anticancer, anti-HIV, antioxidant, antimicrobial, antitubercular, antidiabetic, antimalarial, and anticholinesterase activities .
Biochemical Pathways
The biochemical pathways affected by this compound are diverse, given the broad spectrum of biological activities associated with indole derivatives . For instance, some indole derivatives have been reported to inhibit the influenza A virus , suggesting that they may affect viral replication pathways. Other derivatives have shown anti-inflammatory and analgesic activities , indicating that they may influence pathways related to inflammation and pain perception.
Pharmacokinetics
Indole derivatives are generally known for their broad-spectrum biological activities , which suggests that they may have favorable ADME properties that contribute to their bioavailability.
Result of Action
The molecular and cellular effects of this compound’s action depend on the specific biological activity being exerted. For example, if the compound is acting as an antiviral agent, the result might be the inhibition of viral replication . If it is exerting anti-inflammatory activity, the result could be a reduction in inflammation and pain .
将来の方向性
Indole derivatives have diverse biological activities and also have an immeasurable potential to be explored for newer therapeutic possibilities . The success of computer-aided drug design in the fields of cancer and anti-viral drugs has accelerated in silico studies in antibacterial drug development .
生化学分析
Biochemical Properties
5-Methoxy-1H-indol-4-amine plays a significant role in various biochemical reactions. It interacts with several enzymes, proteins, and other biomolecules. One of the key interactions is with monoamine oxidase (MAO), an enzyme responsible for the breakdown of monoamines. This compound acts as a substrate for MAO, leading to its deamination and subsequent formation of metabolites. Additionally, this compound interacts with serotonin receptors, particularly the 5-HT2A receptor, where it acts as an agonist, influencing neurotransmission and signaling pathways .
Cellular Effects
The effects of this compound on various cell types and cellular processes are profound. In neuronal cells, it modulates neurotransmitter release and uptake, impacting cell signaling pathways and gene expression. This compound has been shown to influence the expression of genes involved in neuroplasticity and synaptic function. In non-neuronal cells, this compound affects cellular metabolism by altering the activity of key metabolic enzymes, leading to changes in cellular energy production and utilization .
Molecular Mechanism
At the molecular level, this compound exerts its effects through several mechanisms. It binds to serotonin receptors, particularly the 5-HT2A receptor, leading to receptor activation and downstream signaling cascades. This binding induces conformational changes in the receptor, triggering intracellular signaling pathways such as the phosphoinositide pathway and the release of intracellular calcium. Additionally, this compound inhibits the activity of monoamine oxidase, preventing the breakdown of monoamines and increasing their availability in the synaptic cleft .
Temporal Effects in Laboratory Settings
In laboratory settings, the effects of this compound change over time. The compound exhibits stability under controlled conditions, but it can undergo degradation when exposed to light and air. Long-term studies have shown that prolonged exposure to this compound can lead to adaptive changes in cellular function, including alterations in receptor sensitivity and gene expression. These changes are often reversible upon removal of the compound .
Dosage Effects in Animal Models
The effects of this compound vary with different dosages in animal models. At low doses, the compound exhibits mild psychoactive effects, influencing behavior and cognition. At higher doses, it can induce significant changes in neurotransmitter levels, leading to altered mood and perception. Toxicity studies have shown that extremely high doses of this compound can cause adverse effects, including neurotoxicity and organ damage .
Metabolic Pathways
This compound is involved in several metabolic pathways. It is primarily metabolized by monoamine oxidase, leading to the formation of metabolites such as 5-methoxyindoleacetic acid. This compound also interacts with cytochrome P450 enzymes, which play a role in its oxidative metabolism. The metabolites of this compound can further participate in various biochemical reactions, influencing metabolic flux and metabolite levels .
Transport and Distribution
Within cells and tissues, this compound is transported and distributed through specific transporters and binding proteins. It is taken up by cells via serotonin transporters and can accumulate in specific tissues, such as the brain and liver. The distribution of this compound is influenced by its lipophilicity, allowing it to cross cell membranes and reach intracellular compartments .
Subcellular Localization
The subcellular localization of this compound is critical for its activity and function. This compound is primarily localized in the cytoplasm, where it interacts with various intracellular targets. It can also be found in the nucleus, where it may influence gene expression by interacting with transcription factors and other nuclear proteins. Post-translational modifications, such as phosphorylation, can affect the localization and activity of this compound, directing it to specific cellular compartments .
準備方法
Synthetic Routes and Reaction Conditions
The synthesis of 5-methoxy-1H-indol-4-amine can be achieved through various methods. One common approach involves the Fischer indole synthesis, where phenylhydrazine reacts with an aldehyde or ketone in the presence of an acid catalyst. For instance, the reaction of 5-methoxy-2-iodoaniline with disilylated alkyne under Larock’s heteroannulation conditions yields 5-methoxy-2,3-disubstituted indole .
Industrial Production Methods
Industrial production of this compound typically involves large-scale synthesis using optimized reaction conditions to ensure high yield and purity. The use of continuous flow reactors and automated systems can enhance the efficiency and scalability of the production process.
化学反応の分析
Types of Reactions
5-Methoxy-1H-indol-4-amine undergoes various chemical reactions, including:
Oxidation: The compound can be oxidized to form corresponding quinones or other oxidized derivatives.
Reduction: Reduction reactions can convert the compound into different reduced forms, such as hydrazines.
Substitution: Electrophilic substitution reactions can introduce various substituents onto the indole ring.
Common Reagents and Conditions
Oxidation: Common oxidizing agents include potassium permanganate and chromium trioxide.
Reduction: Reducing agents such as sodium borohydride and lithium aluminum hydride are often used.
Substitution: Reagents like halogens, nitrating agents, and sulfonating agents are employed under acidic or basic conditions.
Major Products Formed
The major products formed from these reactions depend on the specific reagents and conditions used. For example, oxidation may yield quinones, while substitution reactions can produce halogenated or nitrated indoles.
類似化合物との比較
Similar Compounds
5-Methoxy-2,3-disubstituted indole: Similar in structure but with different substituents at the 2 and 3 positions.
Indole-3-acetic acid: A plant hormone with a carboxylic acid group at the 3-position.
1H-Indole-3-carbaldehyde: An indole derivative with an aldehyde group at the 3-position.
Uniqueness
5-Methoxy-1H-indol-4-amine is unique due to its specific substitution pattern, which imparts distinct chemical and biological properties
特性
IUPAC Name |
5-methoxy-1H-indol-4-amine | |
---|---|---|
Source | PubChem | |
URL | https://pubchem.ncbi.nlm.nih.gov | |
Description | Data deposited in or computed by PubChem | |
InChI |
InChI=1S/C9H10N2O/c1-12-8-3-2-7-6(9(8)10)4-5-11-7/h2-5,11H,10H2,1H3 | |
Source | PubChem | |
URL | https://pubchem.ncbi.nlm.nih.gov | |
Description | Data deposited in or computed by PubChem | |
InChI Key |
YTRLGXQPTYRYKK-UHFFFAOYSA-N | |
Source | PubChem | |
URL | https://pubchem.ncbi.nlm.nih.gov | |
Description | Data deposited in or computed by PubChem | |
Canonical SMILES |
COC1=C(C2=C(C=C1)NC=C2)N | |
Source | PubChem | |
URL | https://pubchem.ncbi.nlm.nih.gov | |
Description | Data deposited in or computed by PubChem | |
Molecular Formula |
C9H10N2O | |
Source | PubChem | |
URL | https://pubchem.ncbi.nlm.nih.gov | |
Description | Data deposited in or computed by PubChem | |
Molecular Weight |
162.19 g/mol | |
Source | PubChem | |
URL | https://pubchem.ncbi.nlm.nih.gov | |
Description | Data deposited in or computed by PubChem | |
Retrosynthesis Analysis
AI-Powered Synthesis Planning: Our tool employs the Template_relevance Pistachio, Template_relevance Bkms_metabolic, Template_relevance Pistachio_ringbreaker, Template_relevance Reaxys, Template_relevance Reaxys_biocatalysis model, leveraging a vast database of chemical reactions to predict feasible synthetic routes.
One-Step Synthesis Focus: Specifically designed for one-step synthesis, it provides concise and direct routes for your target compounds, streamlining the synthesis process.
Accurate Predictions: Utilizing the extensive PISTACHIO, BKMS_METABOLIC, PISTACHIO_RINGBREAKER, REAXYS, REAXYS_BIOCATALYSIS database, our tool offers high-accuracy predictions, reflecting the latest in chemical research and data.
Strategy Settings
Precursor scoring | Relevance Heuristic |
---|---|
Min. plausibility | 0.01 |
Model | Template_relevance |
Template Set | Pistachio/Bkms_metabolic/Pistachio_ringbreaker/Reaxys/Reaxys_biocatalysis |
Top-N result to add to graph | 6 |
Feasible Synthetic Routes
試験管内研究製品の免責事項と情報
BenchChemで提示されるすべての記事および製品情報は、情報提供を目的としています。BenchChemで購入可能な製品は、生体外研究のために特別に設計されています。生体外研究は、ラテン語の "in glass" に由来し、生物体の外で行われる実験を指します。これらの製品は医薬品または薬として分類されておらず、FDAから任何の医療状態、病気、または疾患の予防、治療、または治癒のために承認されていません。これらの製品を人間または動物に体内に導入する形態は、法律により厳格に禁止されています。これらのガイドラインに従うことは、研究と実験において法的および倫理的な基準の遵守を確実にするために重要です。