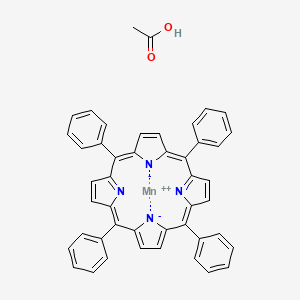
Acetic acid;manganese(2+);5,10,15,20-tetraphenylporphyrin-22,24-diide
説明
Acetic acid, manganese(2+), and 5,10,15,20-tetraphenylporphyrin-22,24-diide (TPP-2) are important components of the chemical synthesis process. Acetic acid is a colorless liquid with a pungent odor and sour taste that is used in a variety of applications, including food preservation and pharmaceuticals. Manganese(2+) is a naturally occurring ion and is used in a variety of industrial processes, such as steel production and water treatment. TPP-2 is a porphyrin derivative that is used in a variety of scientific research applications and is known for its high solubility in aqueous solutions.
科学的研究の応用
Subheading
Oxidation of HydrocarbonsA study by Leduc et al. (1988) describes a biomimetic electrochemical system that uses manganese tetraphenylporphyrin chloride, imidazole, and acetic acid for the activation of dioxygen and oxidation of hydrocarbons. The system successfully epoxidizes alkenes and oxidizes alkanes into alcohols and ketones.
Spectroscopic and AFM Characterization of Manganese Porphyrins
Subheading
Optoelectronic and Surface PropertiesThe work by Fagadar-Cosma et al. (2009) focuses on manganese complexes of tetraphenylporphyrin and their hybrid silica nanomaterials. These materials exhibit bathochromic and hyperchromic effects, orientation of aggregates on surfaces as shown by AFM images, and potential applications in medicine, sensor formulation, and environmental-friendly catalysts for photodegradation of organic compounds.
Biomimetic Decarboxylation of Carboxylic Acids
Subheading
Catalytic DecarboxylationGholamreza and Roxana (2008) conducted a study on the use of manganese(III) meso-tetraphenylporphyrin acetate as a catalyst for the oxidative decarboxylation of carboxylic acids. This method, employing biologically relevant manganese porphyrins, demonstrates efficient carbonyl product formation and highlights the catalyst's selectivity and benign nature (Gholamreza & Roxana, 2008).
Organized Monolayer Films as Supported Oxidation Catalysts
Subheading
Monolayer Films for CatalysisA study by Benitez et al. (2002) explored the preparation of organized monolayer films of manganese tetraphenylporphyrin as supported oxidation catalysts. These films demonstrated enhanced activity in organic oxidations, providing insights into the design of supported molecular catalyst systems.
REDOX and Selective Oxidation Properties
Subheading
Catalytic Oxidation ActivitySaikia and Srinivas (2009) investigated manganese complexes of tetraphenylporphyrin grafted on SBA-15 with different functional groups. The study revealed that the acido-basic properties of the support influenced the oxidation state, redox behavior, and selective oxidation properties of the Mn complexes, offering insights into regio-, stereo-, and chemoselective oxidation (Saikia & Srinivas, 2009).
特性
IUPAC Name |
acetic acid;manganese(2+);5,10,15,20-tetraphenylporphyrin-22,24-diide | |
---|---|---|
Source | PubChem | |
URL | https://pubchem.ncbi.nlm.nih.gov | |
Description | Data deposited in or computed by PubChem | |
InChI |
InChI=1S/C44H28N4.C2H4O2.Mn/c1-5-13-29(14-6-1)41-33-21-23-35(45-33)42(30-15-7-2-8-16-30)37-25-27-39(47-37)44(32-19-11-4-12-20-32)40-28-26-38(48-40)43(31-17-9-3-10-18-31)36-24-22-34(41)46-36;1-2(3)4;/h1-28H;1H3,(H,3,4);/q-2;;+2 | |
Source | PubChem | |
URL | https://pubchem.ncbi.nlm.nih.gov | |
Description | Data deposited in or computed by PubChem | |
InChI Key |
UFMFSWMECMUSKE-UHFFFAOYSA-N | |
Source | PubChem | |
URL | https://pubchem.ncbi.nlm.nih.gov | |
Description | Data deposited in or computed by PubChem | |
Canonical SMILES |
CC(=O)O.C1=CC=C(C=C1)C2=C3C=CC(=C(C4=NC(=C(C5=CC=C([N-]5)C(=C6C=CC2=N6)C7=CC=CC=C7)C8=CC=CC=C8)C=C4)C9=CC=CC=C9)[N-]3.[Mn+2] | |
Source | PubChem | |
URL | https://pubchem.ncbi.nlm.nih.gov | |
Description | Data deposited in or computed by PubChem | |
Molecular Formula |
C46H32MnN4O2 | |
Source | PubChem | |
URL | https://pubchem.ncbi.nlm.nih.gov | |
Description | Data deposited in or computed by PubChem | |
Molecular Weight |
727.7 g/mol | |
Source | PubChem | |
URL | https://pubchem.ncbi.nlm.nih.gov | |
Description | Data deposited in or computed by PubChem | |
Product Name |
Acetic acid;manganese(2+);5,10,15,20-tetraphenylporphyrin-22,24-diide |
Retrosynthesis Analysis
AI-Powered Synthesis Planning: Our tool employs the Template_relevance Pistachio, Template_relevance Bkms_metabolic, Template_relevance Pistachio_ringbreaker, Template_relevance Reaxys, Template_relevance Reaxys_biocatalysis model, leveraging a vast database of chemical reactions to predict feasible synthetic routes.
One-Step Synthesis Focus: Specifically designed for one-step synthesis, it provides concise and direct routes for your target compounds, streamlining the synthesis process.
Accurate Predictions: Utilizing the extensive PISTACHIO, BKMS_METABOLIC, PISTACHIO_RINGBREAKER, REAXYS, REAXYS_BIOCATALYSIS database, our tool offers high-accuracy predictions, reflecting the latest in chemical research and data.
Strategy Settings
Precursor scoring | Relevance Heuristic |
---|---|
Min. plausibility | 0.01 |
Model | Template_relevance |
Template Set | Pistachio/Bkms_metabolic/Pistachio_ringbreaker/Reaxys/Reaxys_biocatalysis |
Top-N result to add to graph | 6 |
Feasible Synthetic Routes
試験管内研究製品の免責事項と情報
BenchChemで提示されるすべての記事および製品情報は、情報提供を目的としています。BenchChemで購入可能な製品は、生体外研究のために特別に設計されています。生体外研究は、ラテン語の "in glass" に由来し、生物体の外で行われる実験を指します。これらの製品は医薬品または薬として分類されておらず、FDAから任何の医療状態、病気、または疾患の予防、治療、または治癒のために承認されていません。これらの製品を人間または動物に体内に導入する形態は、法律により厳格に禁止されています。これらのガイドラインに従うことは、研究と実験において法的および倫理的な基準の遵守を確実にするために重要です。