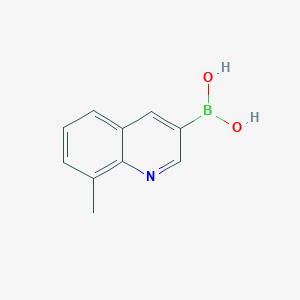
(8-Methylquinolin-3-yl)boronic acid
概要
説明
(8-Methylquinolin-3-yl)boronic acid is a boronic acid derivative with the molecular formula C10H10BNO2 and a molecular weight of 187.01 g/mol . This compound is of significant interest in the field of medicinal chemistry due to its potential therapeutic applications. It is commonly used in research and development for its versatile reactivity and functional group compatibility.
準備方法
Synthetic Routes and Reaction Conditions
The synthesis of (8-Methylquinolin-3-yl)boronic acid typically involves the Suzuki-Miyaura coupling reaction, which is a widely used method for forming carbon-carbon bonds . The reaction generally requires a palladium catalyst, a base, and a boronic acid derivative. The conditions are usually mild and tolerant of various functional groups, making this method highly efficient for synthesizing complex molecules.
Industrial Production Methods
While specific industrial production methods for this compound are not extensively documented, the general approach involves large-scale Suzuki-Miyaura coupling reactions. These reactions are optimized for high yield and purity, often using continuous flow reactors to enhance efficiency and scalability.
化学反応の分析
Suzuki-Miyaura Cross-Coupling Reactions
(8-Methylquinolin-3-yl)boronic acid participates in palladium-catalyzed Suzuki-Miyaura reactions to form biaryl structures, a cornerstone of modern organic synthesis. Key findings include:
-
Catalytic Systems : Optimal yields are achieved using Pd(OAc)₂ with XPhos ligand and KOAc base in toluene at 100°C . Alternative systems like [(dppf)PdCl₂] with Cs₂CO₃ in water/1,4-dioxane (1:3) at 100°C also demonstrate efficacy .
-
Substrate Scope : The compound couples with aryl halides (e.g., 4-bromophenoxyquinoline derivatives) to yield biaryls (e.g., 5a–5o ), with yields ranging from 55% to 75% depending on substituents .
-
Mechanistic Insight : Transmetalation proceeds via a boronate intermediate, where the boron atom coordinates to palladium, followed by reductive elimination .
Table 1: Representative Suzuki-Miyaura Reaction Conditions
Substrate | Catalyst System | Base | Solvent | Yield (%) | Source |
---|---|---|---|---|---|
4-Bromophenoxyquinoline | [(dppf)PdCl₂] (5 mol %) | Cs₂CO₃ | H₂O/1,4-dioxane | 63–75 | |
Aryl Halides | Pd(OAc)₂/XPhos | KOAc | Toluene | 35–45 |
Formation of Boronate Esters and Derivatives
The boronic acid group enables conversion into stabilized boronates:
-
Trifluoroborate Salts : Treatment with KHF₂ in MeOH yields potassium trifluoroborates (e.g., 10 ), which enhance stability and solubility for storage .
-
Oxaboroles : Reaction with diols under dehydrating conditions forms cyclic boronate esters, useful in sensor design .
Complexation with Biological Molecules
The Lewis acidic boron atom forms reversible complexes with biomolecules:
-
Sugar Binding : Exhibits strong binding to D-fructose (Keq = 120 M⁻¹ at pH 7.4) via boronate ester formation, outperforming phenylboronic acid analogs .
-
Protein Interactions : Coordinates with electron-rich residues (e.g., Thr347, Glu353) in breast cancer protein 3ERT, as shown by molecular docking studies .
Substituent Effects on Reactivity
The 8-methyl group on the quinoline ring influences electronic and steric properties:
-
Electronic Modulation : The methyl group donates electron density to the quinoline ring, reducing boron’s Lewis acidity compared to fluoro-substituted analogs (e.g., 8-fluoroquinolin-3-yl boronic acid) .
-
Steric Hindrance : Ortho-substituted derivatives (e.g., 2-methylphenylboronic acid) show reduced reactivity in cross-couplings due to hindered Pd-B interaction .
Mechanistic Studies and Side Reactions
科学的研究の応用
Medicinal Chemistry
1.1 Pharmaceutical Development
(8-Methylquinolin-3-yl)boronic acid serves as a versatile building block for synthesizing novel drug candidates. The quinoline core is recognized as a privileged scaffold in medicinal chemistry, often associated with various biological activities. The incorporation of the boronic acid group facilitates Suzuki-Miyaura coupling reactions, enabling the construction of complex molecules that may exhibit therapeutic properties.
1.2 Biological Activity
Research indicates that this compound can interact with biological targets through boron coordination chemistry. This interaction is particularly relevant in cancer therapy, where boronic acids are explored as inhibitors for specific enzymes involved in metabolic pathways. Notably, studies have shown that this compound can form stable complexes with biomolecules, driven by the Lewis acidity of the boron atom, which allows coordination with electron-rich sites on proteins or nucleic acids.
Case Study: Inhibition of Homeodomain Interacting Protein Kinase 2 (HIPK2)
A recent study demonstrated the potential of borylated quinolines, including derivatives of this compound, as inhibitors of HIPK2, a key regulator in kidney fibrosis progression. The research highlighted a Pd-catalyzed borylation method that efficiently synthesized these compounds, which were further evaluated for their pharmacological activity .
Organic Synthesis
2.1 Cross-Coupling Reactions
The boronic acid functional group in this compound enables its participation in various cross-coupling reactions, which are essential for constructing complex organic molecules. These reactions are pivotal in synthesizing pharmaceuticals and agrochemicals .
2.2 Synthesis Methodologies
Several synthetic routes have been developed for this compound, including:
These methodologies highlight the compound's utility in organic synthesis and its role as a precursor for more complex structures.
Material Science
3.1 Sensor Development
The unique properties of boronic acids allow this compound to be utilized in developing self-assembling materials and sensors. Its ability to form reversible covalent bonds with certain functional groups makes it suitable for creating responsive materials that can detect specific analytes.
作用機序
The mechanism of action of (8-Methylquinolin-3-yl)boronic acid involves its interaction with specific molecular targets. In medicinal chemistry, boronic acids are known to inhibit enzymes by forming reversible covalent bonds with active site residues. This interaction can modulate the activity of the enzyme, leading to therapeutic effects. The exact molecular pathways and targets depend on the specific application and context of use.
類似化合物との比較
Similar Compounds
- (8-Methylquinolin-2-yl)boronic acid
- (8-Methylquinolin-4-yl)boronic acid
- (8-Methylquinolin-5-yl)boronic acid
Uniqueness
(8-Methylquinolin-3-yl)boronic acid is unique due to its specific substitution pattern on the quinoline ring, which can influence its reactivity and interaction with biological targets. This uniqueness makes it a valuable compound for developing selective inhibitors and other therapeutic agents .
生物活性
(8-Methylquinolin-3-yl)boronic acid is a compound that has garnered attention in medicinal chemistry due to its diverse biological activities. This article explores its biological activity, focusing on anticancer, antibacterial, and potential therapeutic applications, supported by data tables and relevant research findings.
Overview of Boronic Acids
Boronic acids, including this compound, are known for their ability to form reversible covalent bonds with diols and have been utilized in various medicinal applications. Their unique chemical properties enable them to act as enzyme inhibitors, particularly in cancer therapy and antibacterial treatments.
Anticancer Activity
Recent studies have demonstrated that this compound exhibits significant anticancer properties.
The mechanism of action primarily involves the inhibition of proteasome activity, which is crucial for regulating the cell cycle and apoptosis. For instance, boronic acid derivatives have been shown to stop cell cycle progression at the G2/M phase in cancer cells, leading to growth inhibition .
Case Studies
- Inhibitory Potency : In vitro studies revealed that compounds similar to this compound possess IC50 values ranging from 6 nM to 8 nM against various cancer cell lines, indicating potent inhibitory effects on tumor growth .
- Pharmacokinetics : Pharmacokinetic studies in animal models suggest that these compounds can be administered intravenously with promising bioavailability, although further optimization is needed to enhance targeting efficacy .
Antibacterial Activity
Boronic acids have also been explored for their antibacterial properties, particularly against resistant bacterial strains.
The antibacterial activity is attributed to the ability of boronic acids to inhibit β-lactamases, enzymes that confer resistance to β-lactam antibiotics. The interaction occurs through covalent binding to serine residues within the active site of these enzymes .
Research Findings
- Inhibitory Constants : Compounds derived from this compound demonstrate low inhibitory constants (Ki) against class C β-lactamases, with values as low as 0.004 µM reported for highly resistant strains .
- Biofilm Inhibition : Recent studies have highlighted the potential of these compounds in preventing biofilm formation by Pseudomonas aeruginosa, a common pathogen in nosocomial infections .
Data Summary Table
Future Directions
The ongoing research into this compound suggests several avenues for future exploration:
- Lead Optimization : Further structural modifications may enhance solubility and target specificity for both anticancer and antibacterial applications.
- Combination Therapies : Investigating the efficacy of this compound in combination with existing therapies could yield synergistic effects, particularly in resistant strains or advanced-stage cancers.
- Clinical Trials : Transitioning from preclinical studies to clinical trials will be crucial for assessing the therapeutic potential and safety profile of this compound.
Q & A
Q. What synthetic strategies are effective for optimizing the yield of (8-Methylquinolin-3-yl)boronic acid derivatives?
Basic
The synthesis of quinoline-derived boronic acids often involves coupling reactions, purification via column chromatography, and characterization using NMR and mass spectrometry. For example, in the synthesis of structurally similar bromo-quinoline derivatives, THF and methylamine have been used as solvents and reactants, followed by extraction with EtOAc and purification with MeOH:DCM (1:99) to achieve high yields (>95%) . Key factors include solvent choice, reaction time, and chromatographic conditions.
Q. How do thermodynamic and kinetic properties of boronic acid-diol binding influence chemosensor design?
Advanced
The binding affinity (thermodynamic) and reaction rate (kinetic) of boronic acids with diols (e.g., sugars) are critical for real-time sensing applications. Stopped-flow kinetic studies reveal that kon values follow the order D-fructose > D-tagatose > D-mannose > D-glucose, correlating with thermodynamic stability . This suggests that the "on" rate (kon) is a dominant factor in binding constants. Researchers must balance pH (physiological pH preferred), diol structure, and steric effects when designing sensors for rapid equilibrium attainment.
Q. What analytical challenges arise in mass spectrometry (MS) characterization of boronic acid-containing peptides, and how can they be mitigated?
Advanced
Free boronic acids undergo dehydration/trimerization, complicating MS analysis. Derivatization with diols (e.g., pinacol) to form boronic esters prevents trimerization. For MALDI-MS, 2,5-dihydroxybenzoic acid (DHB) serves as both matrix and derivatizing agent, enabling efficient in situ esterification and sequencing of branched peptides with up to five boronic acid groups . This method reduces sample preparation time and improves signal clarity.
Q. How can boronic acid-functionalized polymers be tailored for glucose-responsive applications?
Basic
Boronic acid polymers (e.g., poly(3-acrylamidophenylboronic acid)) exploit reversible diol binding to modulate solubility. Under acidic/neutral conditions, boroxine crosslinking renders the polymer insoluble, while glucose competes for binding, increasing solubility . Researchers should optimize polymer backbone flexibility, boronic acid density, and buffer pH to achieve glucose-responsive behavior suitable for drug delivery or sensing.
Q. What methodologies are used to assess boronic acid interactions with glycoproteins in surface-based assays?
Advanced
Surface plasmon resonance (SPR) with boronic acid-functionalized dextran substrates reveals binding dependencies on terminal saccharide moieties. For example, AECPBA surfaces show higher affinity for glycoproteins with exposed cis-diols (e.g., RNAse B) but require buffer optimization (e.g., borate buffer at pH 8.5) to minimize non-specific interactions . Secondary interactions (e.g., electrostatic) must be controlled through ionic strength adjustments or competitive eluents.
Q. How do boronic acids enhance anticancer drug design, and what structural features correlate with activity?
Advanced
Boronic acids act as reversible covalent inhibitors, targeting enzymes like the proteasome (e.g., Bortezomib) . Structure-activity studies on cis-stilbene analogs show that replacing hydroxyl groups with boronic acids enhances tubulin polymerization inhibition (IC50 ~21–22 μM) and apoptosis induction . Key features include planar aromatic systems and boronic acid positioning for optimal target engagement.
Q. Can boronic acid-diol binding be dynamically controlled for smart materials?
Advanced
Azobenzene-boronic acid conjugates enable light-controlled binding. E→Z isomerization with red light enhances diol affinity by 20-fold via steric and electronic modulation . Applications include photoswitchable hydrogels, where crosslinking stiffness is tuned by wavelength-dependent boronic ester formation. Researchers should prioritize azobenzene substituents (e.g., 2,6-dimethoxy groups) to maximize binding contrast between isomers.
Q. What LC-MS/MS strategies are effective for detecting trace boronic acid impurities in pharmaceuticals?
Advanced
Triple quadrupole LC-MS/MS in MRM mode allows sensitive detection of underivatized boronic acids (e.g., in Lumacaftor drug substances) without time-consuming derivatization . Key parameters include ionization mode (ESI+), collision energy optimization, and column selection (C18 with acidic mobile phases). Method validation should address linearity (0.1–10 ppm) and recovery rates (>90%).
Q. How do secondary interactions affect the selectivity of boronic acid-based glycoprotein capture systems?
Advanced
Non-specific interactions (e.g., electrostatic) can reduce selectivity. For AECPBA surfaces, secondary binding with non-glycosylated proteins like avidin is mitigated by adjusting buffer ionic strength or using competitive eluents (e.g., sorbitol) . Researchers must characterize binding thermodynamics (SPR or ITC) and employ blocking agents (e.g., BSA) to improve specificity.
Q. What in vitro models are suitable for evaluating boronic acid derivatives as anticancer agents?
Basic
Glioblastoma cell lines (e.g., U87) are common for cytotoxicity screening. Compounds are tested via MTT assays, with IC50 values compared to controls (e.g., combretastatin A-4). FACScan analysis can confirm apoptosis induction (e.g., Jurkat cells treated with 10⁻⁸ M boronic acid derivatives) . Dose-response curves and COMPARE analysis (NCI-60 panel) help identify unique mechanisms vs. known drugs.
特性
IUPAC Name |
(8-methylquinolin-3-yl)boronic acid | |
---|---|---|
Source | PubChem | |
URL | https://pubchem.ncbi.nlm.nih.gov | |
Description | Data deposited in or computed by PubChem | |
InChI |
InChI=1S/C10H10BNO2/c1-7-3-2-4-8-5-9(11(13)14)6-12-10(7)8/h2-6,13-14H,1H3 | |
Source | PubChem | |
URL | https://pubchem.ncbi.nlm.nih.gov | |
Description | Data deposited in or computed by PubChem | |
InChI Key |
QNMGKSYRNLBGNT-UHFFFAOYSA-N | |
Source | PubChem | |
URL | https://pubchem.ncbi.nlm.nih.gov | |
Description | Data deposited in or computed by PubChem | |
Canonical SMILES |
B(C1=CC2=CC=CC(=C2N=C1)C)(O)O | |
Source | PubChem | |
URL | https://pubchem.ncbi.nlm.nih.gov | |
Description | Data deposited in or computed by PubChem | |
Molecular Formula |
C10H10BNO2 | |
Source | PubChem | |
URL | https://pubchem.ncbi.nlm.nih.gov | |
Description | Data deposited in or computed by PubChem | |
Molecular Weight |
187.00 g/mol | |
Source | PubChem | |
URL | https://pubchem.ncbi.nlm.nih.gov | |
Description | Data deposited in or computed by PubChem | |
CAS No. |
1370040-72-4 | |
Record name | (8-methylquinolin-3-yl)boronic acid | |
Source | European Chemicals Agency (ECHA) | |
URL | https://echa.europa.eu/information-on-chemicals | |
Description | The European Chemicals Agency (ECHA) is an agency of the European Union which is the driving force among regulatory authorities in implementing the EU's groundbreaking chemicals legislation for the benefit of human health and the environment as well as for innovation and competitiveness. | |
Explanation | Use of the information, documents and data from the ECHA website is subject to the terms and conditions of this Legal Notice, and subject to other binding limitations provided for under applicable law, the information, documents and data made available on the ECHA website may be reproduced, distributed and/or used, totally or in part, for non-commercial purposes provided that ECHA is acknowledged as the source: "Source: European Chemicals Agency, http://echa.europa.eu/". Such acknowledgement must be included in each copy of the material. ECHA permits and encourages organisations and individuals to create links to the ECHA website under the following cumulative conditions: Links can only be made to webpages that provide a link to the Legal Notice page. | |
Retrosynthesis Analysis
AI-Powered Synthesis Planning: Our tool employs the Template_relevance Pistachio, Template_relevance Bkms_metabolic, Template_relevance Pistachio_ringbreaker, Template_relevance Reaxys, Template_relevance Reaxys_biocatalysis model, leveraging a vast database of chemical reactions to predict feasible synthetic routes.
One-Step Synthesis Focus: Specifically designed for one-step synthesis, it provides concise and direct routes for your target compounds, streamlining the synthesis process.
Accurate Predictions: Utilizing the extensive PISTACHIO, BKMS_METABOLIC, PISTACHIO_RINGBREAKER, REAXYS, REAXYS_BIOCATALYSIS database, our tool offers high-accuracy predictions, reflecting the latest in chemical research and data.
Strategy Settings
Precursor scoring | Relevance Heuristic |
---|---|
Min. plausibility | 0.01 |
Model | Template_relevance |
Template Set | Pistachio/Bkms_metabolic/Pistachio_ringbreaker/Reaxys/Reaxys_biocatalysis |
Top-N result to add to graph | 6 |
Feasible Synthetic Routes
試験管内研究製品の免責事項と情報
BenchChemで提示されるすべての記事および製品情報は、情報提供を目的としています。BenchChemで購入可能な製品は、生体外研究のために特別に設計されています。生体外研究は、ラテン語の "in glass" に由来し、生物体の外で行われる実験を指します。これらの製品は医薬品または薬として分類されておらず、FDAから任何の医療状態、病気、または疾患の予防、治療、または治癒のために承認されていません。これらの製品を人間または動物に体内に導入する形態は、法律により厳格に禁止されています。これらのガイドラインに従うことは、研究と実験において法的および倫理的な基準の遵守を確実にするために重要です。